Abstract
Although azomethine ylides have been fully exploited as versatile reactive intermediates in dipolar cycloaddition reactions to construct a variety of heterocyclic compounds involving a nitrogen atom, little is known about their structural and electronic properties. Here a method is developed for the preparation, isolation and characterization of a stable 2-azaphenalenyl based azomethine ylide. N-Phenyl-5,8-di-t-butyl-2-azaphenalenyl cannot be isolated because it undergoes rapid dimerization by C–C bond formation at the 1 and 3 positions. In contrast, sterically bulkier N-2,6-di(isopropyl)phenyl-5,8-di-t-butyl-2-azaphenalenyl can be generated and isolated as deep green crystals under deoxygenated conditions. X-ray crystal analysis of this stable azomethine ylide reveals that its azaphenalenyl skeleton has very small bond alternation and structural deformation. The results of spectroscopic and electrochemical theoretical studies indicate that N-2,6-di(isopropyl)phenyl-5,8-di-t-butyl-2-azaphenalenyl has electronic features that are similar to those of phenalenyl anion and it possesses an extremely high HOMO energy.
Similar content being viewed by others
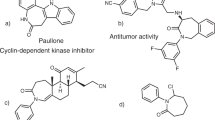
Introduction
Since the first description in 1862 by Strecker1, azomethine ylides have been extensively used in organic synthesis as 3-atom, 4π-electron components of 1,3-dipolar cycloaddition reactions with dipolarophiles. Regardless of the fact that the reactivity and methods for generation of these important reactive intermediates have been investigated intensively2,3,4, little is known about their detailed structural and electronic properties. Carbonyl-stabilized pyridinium ylide 1 as an azomethine ylide vinylog was synthesized in 19343,5. However, its electronic and structural properties were not elucidated, and it does not have the ability to undergo 1,3-dipolar cycloaddition reactions with dipolarophiles. Azaphenalenes, 14π electron containing isoelectronic analogs of phenalenyl anion 2, are composed of the four structural isomers displayed in Fig. 1a. The isomers includes 1-azaphenalene 36 and 9b-azaphenalene (cycl[3.3.3]azine) 47, which have been synthesized, and N-substituted-2-azaphenalenyl (benzo[de]isoquinolinium-1-ide) 58,9 and 9a-azaphenalenyl 6, which serve as highly reactive azomethine ylides intermediates (Fig. 1b and Supplementary Fig. 1). Dibenzo derivatives of 610,11,12,13,14,15 were explored earlier by Beljonne, Feng and Müllen, who also reported their absorption spectral properties10. N-Substituted 2-azaphenalenyl derivatives 5 can be structurally visualized in three resonance forms including azomethine ylide A, azaphenalenyl anion B and biradical C (Fig. 1c).
The effort described here is aimed at the isolation, and structural and electronic characterization of an azomethine ylide. In order to incorporate features that would lead to stabilization of these typically transient species, we design N-substituted 2-azaphenalenyl 7a and 7b, which contain phenyl and 2,6-di(isopropyl)phenyl groups on nitrogen, as well as bulky tert-butyl groups at the 5 and 8 positions. Below, we describe the method employed to isolate the aerobically unstable azomethine ylide 7b as a green solid and, its structural and electronic characterization.
Results
Synthesis and reactions
For preparation of the respective N-phenyl- and 2,6-di(isopropyl)phenyl-2-azaphenalenyl ylides 7a and 7b, we chose to use a protocol involving base induced deprotonation of the corresponding iminium salts 8a and 8b. The route began with triphenylcarbenium tetrafluoroborate (Ph3CBF4) promoted oxidation of the respective amines 9a and 9b (Fig. 2a and Supplementary Fig. 2). Reaction of 8a with triethylamine (Et3N) in THF in the presence of N-phenyl maleimide (NPMI) produced the dipolar cycloaddition adduct 10 in 66% yield (Fig. 2b). This observation demonstrates that the corresponding azomethine ylide 7a is generated as a reactive intermediate by Et3N induced deprotonation of 8a. Additionally, reaction of 7a with the as a versatile synthetic intermediate16,17,18,19 N-(2-ethylhexyl)acenphthylene-5,6-dicarboxylimide 11 was examined. Under similar conditions, this reaction produces the corresponding cycloadduct 12 in 31% yield (Fig. 2c). Interestingly, treatment of 8a in the filter paper component of a Soxhlet extractor with hexane-Et3N vapor led to formation of an amber solid, which upon chromatographic purification produced the colorless dimer initially identified as the dimeric adduct 13 (51%) (Fig. 2d). The results of X-ray crystallographic analysis showed that 13 is comprised of two units of 7a joined to one another in an anti-manner via C–C bonds at the 1 and 3 positions of each (Fig. 2e, Supplementary Figs. 3 and 4, and Supplementary Table 1).
This unusual dimerization process20 suggests that 7a has both biradical and azomethine ylide character. Actually, a theoretical calculation on 5a at the LC-UBLYP(μ = 0.33 bohr–1)/6–311 G* level indicated that it has small biradical character (y = 0.020) (Supplementary Fig. 5 and Supplementary Table 2). The estimated biradical index of 5a is small, but it is larger than that of 5b (R = 2,6-di(isopropyl)phenyl; y = 0.000). Thus, it is possible that conjugation of the N-phenyl group and main core π-system gives 7a the biradical character needed to drive the dimerization process.
We believed that it would be possible to prevent this dimerization reaction by replacing the N-phenyl group in 7a with the sterically more bulky 2,6-di(isopropyl)phenyl group. To assess this proposal, iminium salt 8b was prepared from amine 9b through Ph3CBF4 induced oxidation followed by Et3N vapor promoted deprotonation in the manner employed to form 7a (Fig. 2a). This process formed a green solution of the ylide 7b, which yielded a green solid upon evaporation. Owing to the high reactivity of 7b with oxygen, the green color of both the solution and solid immediately changed to yellow under aerobic conditions (Supplementary Fig. 6). To avoid this problem and simplify the procedure, we used a method to form 7b by reaction of iminium salt 8b with sodium hydride (NaH) in THF in a nitrogen-filled glove box. In this event, ylide 7b was generated as a green crystalline solid quantitatively.
Absorption spectra
Ylide 7b was characterized by using UV-vis, FT-IR, 1H-NMR and 13C-NMR spectroscopy, and high resolution mass spectrometry (HRMS), and finally by using X-ray crystallographic analysis. The absorption spectra of 7b and its precursor 8b in dichloromethane (DCM) are displayed in Fig. 3. The spectrum of 7b is comprised of two bands in the 300–450 and 500–800 nm regions, the latter of which has vibronic structure with absorption maxima (λmax (log ε)) values of 614 (2.24), 665 (2.34), and 727 nm (2.19). The results of TD-DFT calculations on the analog 5b indicate that the 632 nm band corresponds to a HOMO‒LUMO transition (Supplementary Fig. 7 and Supplementary Table 3). While 8b in DCM exhibits intense fluorescence with a maximum (λFL) at 529 nm and a quantum yield (ϕ) of 0.60 (Supplementary Fig. 8), the corresponding ylide 7b is non-fluorescent.
Crystallographic analysis and HOMA values
X-ray crystallography was carried out on a single crystal of 7b (monoclinic P21/c space group) obtained using hexane as a solvent. Analysis of the molecular structure shows that the 2-azaphenalenyl system in 7b possesses a highly planar structure (Fig. 4). The dihedral angle between the azaphenalenyl plane and that of the N-aryl ring is 84.7°, indicating that the N-phenyl substituent only minimally perturbs the electronic properties of 7b (Supplementary Figs. 9 and 10, and Supplementary Table 4). The two C‒N bond lengths in the crystal structure of 7b are nearly identical (1.3492(18) and 1.3516(19) Å), and the average (1.350 Å) is comparable to the C–N bonds (1.34 Å) in N,N’-dibenzyl-dibenzodiazapyrene dication 1421. While from a structural perspective the C‒N‒C unit of 7b is similar to that in the pyridinium ring of 14, the neighboring NC‒C bonds (1.420(2) and 1.421(2) Å) are slightly longer than the corresponding NC‒C bonds in 14 (1.39 Å). Moreover, the C‒N‒C bond angle (123.9(1)˚) in 7b is slightly different than that in a regular hexagon framework because of the shorter nature of C–N vs C-C bonds. All sp2 carbon bond angles in 7b are between 117.4˚ and 123.3˚ (Supplementary Fig. 10). The harmonic oscillator model of aromaticity (HOMA)22,23 value of the pyridine ring in 7b of 0.62 indicates that it has aromatic character (Fig. 4). On the other hand, the benzene rings of the naphthalene moiety in 7b display a small degree of bond alternation (HOMA = 0.85 and 0.79) and are larger than that of naphthalene itself (0.70)24.
NMR analysis and NICS(1) values
The 1H- and 13C-NMR spectra of 7b in THF-d8 (Supplementary Figs. 11 and 12) contain sharp signals, suggesting that, as is predicted based on theoretical calculations, this substance has negligible biradical character. 2D NMR (HSBC, HSQC and NOESY) spectral analyses enable unambiguous assignment of all protons and carbons in 7b (Supplementary Figs. 13‒16). Selected chemical shift data and nucleus-independent chemical shifts (NICS) (1)25 values of 7b together with those of 226,27, 428 and 5b are listed in Fig. 5. Inspection of the 13C-NMR chemical shifts indicate that the negative charge in 7b is delocalized over the entire molecule in a manner that is comparable to that in the phenalenyl anion 2. Theoretical calculations at the GIAO-HF/6–311 + G*//RB3LYP-D3/6–311 G* level indicate that the respective NICS(1) values of the pyridine A and benzene B rings in 5b are +2.75 and −6.21, indicating that the pyridine ring is slightly paratropic and the benzene rings of the naphthalene moiety are weakly diatropic (Fig. 5). Taking into account the negative charge distribution, the observed chemical shifts suggest that 7b is a weak diatropic species and, as a result, it can be regarded as a phenalenyl anion perturbed by the presence of a positively charged nitrogen atom.
Electrochemical properties
The electrochemical properties of 7b were evaluated by using cyclic voltammetry (CV) (Fig. 6 and Supplementary Fig. 17). The CV of 7b in DCM contains three redox waves associated with irreversible oxidation (+0.56 V), reversible oxidation (−0.68 V) and irreversible reduction (−2.06 V), which correspond to the following transformations: 7b2+ ← 7b•+ ⇄ 7b → 7b•‒ (Table 1). The E1ox value (−0.68 V) is extremely high for a neutral compound especially when considering the fact that the typical electron donor tetrathiafulvalene (TTF)29 has an oxidation potential of +0.37 V. Moreover, the high E1ox value of 7b explains its high reactivity toward oxygen. Finally, the HOMO‒LUMO energy gap, estimated using redox potential data) is comparable to that of absorption edge of 7b (1.51 eV/823 nm) (Supplementary Fig. 7).
Discussion
In the effort described above, N-phenyl- and N-2,6-di(isopropyl)phenyl-2-azaphenalenyl, 7a and 7b, were generated from the corresponding iminium ions 8a and 8b by treatment with gaseous Et3N. The phenyl derivative 7a is a highly reactive species that, in the absence of a dipolarophile, undergoes ready dimerization by C–C bond formation at the 1 and 3 positions. This finding is in good accord with the results of theoretical calculations that indicate that 5a possesses slight biradical character. In contrast, 7b containing a bulky N-substituent is more stable and can be isolated under anaerobic conditions. Because the use of substituents for further electronic stabilization could result in loss of azomethine ylide features, like that occurring with 1, 7b should be a good model for elucidation of the electronic and structural features of azomethine ylides. X-ray analysis of 7b reveals that the structural features corresponding to the azomethine ylide unit are similar to those of the corresponding region of diazapyrene dication 14. The characteristics of 7b, evaluated by using spectroscopy, indicate that it has electronic features that are similar to those of phenalenyl anion 2. The results of HOMA and NICS(1) calculations also support this conclusion. Finally, the high reactivity of 7b with molecular oxygen is a consequence of its high oxidation potential associated with a HOMO energy.
The results described above open the way for construction of new compounds bearing the N-substituted 2-azaphenalenyl core structure. For example, it is possible that appropriate functionalization can be used to tune the electronic properties of the 2-azaphenalenyl system and to produce electron donor systems with high thermal stabilities. Moreover, transition metal complexes comprised of N-substituted 2-azaphenalenyl ligands would be intriguing from both physicochemical and synthetic points of view30.
Methods
General
All reactions of air- or moisture-sensitive compounds were carried out in a dry vessel under a positive pressure of nitrogen. Air- and moisture-sensitive liquids and solutions were transferred via syringe. Analytical thin-layer chromatography was performed using glass plates pre-coated with Merck Art. 7730 Kiesel-gel 60 GF-254. Thin layer chromatography plates were visualized by exposure to UV light. Organic solutions were concentrated by using rotary evaporation at ca. 15 Torr obtained with a diaphragm pump. Column chromatography was performed with Merck Kiesel-gel 60. All reagents were commercially available and used without further purification unless otherwise noted. THF was purchased from Wako Chemical Co. and distilled from lithium aluminum hydride at 760 Torr under a nitrogen atmosphere before use.
Melting points were recorded on a Yanaco MP–S3 apparatus and reported uncorrected. Positive FAB and EI mass spectra were recorded on a JEOL JMS-700 and a Shimadzu GCMS-QP2010 Ultra, respectively. High-resolution mass spectra were obtained using an Applied Biosystem Japan Ltd. 1H and 13C NMR spectra of all compounds except 7b and 12 were recorded using a Bruker-Biospin DRX-500 spectrometer. The NMR spectral data were measured at 20 °C. IR spectra of all compounds except 7b and 12 were obtained using a Shimadzu FTIR-8400 spectrometer. NMR spectra of 7b and 12 were recorded using JEOL-AL400 (400 MHz for 1H, and 100 MHz for 13C) and Bruker AVANCE III spectrometers (600 MHz for 1H, and 150 MHz for 13C) with TMS as an internal standard. 19F and 11B NMR spectra of 8a and 8b were recorded using a JEOL-AL400 spectrometer (126.9 MHz for 11B, and 372.4 MHz for 19F) with BF3 Et2O as an external standard. UV–vis and fluorescence spectra of 8a and 8b in DCM were recorded using a JASCO V650 and a SHIMADZU RF-5300PC spectrophotometer, respectively. Quantum yields for solution state fluorescence (ΦSN) of 8a and 8b were determined using 9,10-diphenylanthracene (ΦSN = 0.86)31 in DCM as an actinometer. 1H and 13C NMR resonances of 7b were assigned using HSQC, HSBC, NOESY, and 13C off-resonance techniques. UV-vis-NIR spectra of 7b and 12 were recorded using a SHIMAZU UV-3600 spectrophotometer. The IR spectrum of 7b and 12 was recorded as a solid in a KBr pellet using a JASCO FT/IR 6200 spectrophotometer. Cyclic voltammetric measurements of 7b was performed using an ALS-600C electrochemical analyzer with a glassy carbon working, Pt counter, and Ag/AgNO3 reference electrodes at room temperature in DCM containing 0.1 M Bu4NClO4 as the supporting electrolyte. DFT calculations were carried out using the Gaussian 09 program package32.
Synthesis of 9a and 9b
The naphthopiperidine derivative 9a and 9b were synthesized starting with 3,6-di-tert-butyl-1,8-naphthalenedicarboxylic acid anhydride33 through sequential reduction with lithium aluminum hydride, bromination with boron tribromide, and respective reactions with aniline and 2,6-diisopropylaniline. Full synthetic details are given in the Supplementary Methods.
N-Phenyl-5,8-di-tert-butyl-1H-benz[de]isoquinolinium tetrafluoroborate 8a
A mixture of 9a (0.10 g, 0.29 mmol) and Ph3CBF4 (0.10 g, 0.36 mmol) in dry-acetonitrile (2 mL) was stirred at reflux for 2 h. After cooling to rt, the mixture was diluted with diethyl ether (Et2O) to afford 8a (0.11 g, 0.26 mmol) as an orange precipitate in 94% yield. Decomp. 242−243 °C; 1H NMR (500 MHz, CDCl3): δ 9.45 (s, 1 H), 8.49 (s, 1 H), 8.15 (s, 1 H), 7.80 (m, Jo,m = 8.0 Hz, 2 H, o-H of Ph), 7.77 (s, 1 H), 7.61 (s, 1 H), 7.55 (m, Jo,p = 7.7 Hz, 2 H, m-H of Ph), 7.48 (m, Jm,p = 7.4 Hz, 1 H, p-H of Ph), 5.92 (s, 2 H), 1.45 (s, 9 H), 1.41 (s, 9 H); 13C NMR (126 MHz, CDCl3) δ 166.7, 152.4, 151.5, 141.9, 137.7, 135.6, 132.2, 131.6, 130.7, 124.3, 124.1, 123.1, 122.1, 121.8, 56.0, 35.31, 35.28, 31.1, 30.8 ppm; 11B{1H} NMR (127 MHz, CDCl3) δ −0.90 ppm; 19F NMR (372 MHz, CDCl3) δ 1.70 ppm; IR (KBr): ν 2956 (s), 2908 (m), 2871 (m), 1635 (m), 1608 (m), 1581 (m), 1524 (m), 1469 (m), 1377 (m), 1243 (m), 1083 (s), 758 (m) cm−1; UV/Vis (DCM): λmax (log ε) 287 (3.90), 437 (3.83) nm; FL (DCM): λFL 555 nm (ΦSN = 0.11); HRMS (FAB, NBA) m/z: calcd. for [C26H30N+]: 356.2373; found: 356.2377.
N-2,6-Di(isopropyl)phenyl-5,8-di-tert-butyl-1H-benz[de]isoquinolinium tetrafluoroborate 8b
A mixture of 9b (0.11 g, 0.24 mmol) and Ph3CBF4 (0.093 g, 0.28 mmol) in dry-acetonitrile (2 mL) was stirred at reflux for 2 h. After cooling to rt, the mixture was concentrated in vacuo. The resulting solid was dissolved in ethyl acetate and diluted with hexane to afford 8b (0.114 g, 0.22 mmol) a yellow precipitate in 90% yield. Decomp. 239−240 °C; 1H NMR (500 MHz, CDCl3): δ 9.47 (s, 1 H), 8.73 (s, 1 H), 8.20 (s, 1 H), 7.83 (s, 1 H), 7.56 (t, J = 7.6 Hz, 1 H), 7.55 (s, 1 H), 7.37 (d, J = 7.9 Hz, 2 H), 5.55 (s, 1 H), 2.94 (sep, J = 6.5 Hz, 2 H), 1.47 (s, 9 H), 1.42 (s, 9 H), 1.34 (d, J = 6.5 Hz, 6 H), 1.32 (d, J = 6.5 Hz, 6 H); 13C NMR (126 MHz, CDCl3) δ 171.2, 152.4, 152.1, 142.9, 138.9, 137.4, 135.8, 132.4, 131.9, 128.3, 125.7, 123.7, 123.6, 122.1, 120.9, 58.6, 35.4, 35.3, 31.1, 30.8, 28.9, 24.7, 24.2 ppm; 11B{1H} NMR (127 MHz, CDCl3) δ −0.99 ppm; 19F NMR (372 MHz, CDCl3) δ 1.93 ppm; IR (KBr): ν 2959 (s), 2870 (m), 1644 (m), 1614 (m), 1520 (m), 1464 (m), 1377 (m), 1281 (m), 1241 (m), 1218 (m), 1185 (m), 1047 (s) cm−1; UV/Vis (DCM): λmax (log ε) 269 (4.10), 433 (3.86) nm; FL (DCM): λFL 529 nm (ΦSN = 0.60); HRMS (FAB, NBA) m/z: calcd. for [C32H42N+]: 440.3312; found: 440.3319.
N-Phenylmaleimide adduct 10
A mixture of 8a (100 mg, 0.30 mmol), Et3N (0.15 mL, 1.1 mmol) and NPMI (66 mg, 0.38 mmol) in dry-acetonitrile (3 mL) was stirred at reflux under N2 for overnight. Concentration in vácuo gave a residue that was subjected to column chromatography on silica gel (elution with 2:1 DCM:ethyl acetate) to afford 10 (54 mg, 0.13 mmol) as a colorless solid in 66% yield. M.p. 249−250 °C; 1H NMR (500 MHz, CDCl3): δ 7.54 (d, J = 1.7 Hz, 2 H), 7.43 (d, J = 1.7 Hz, 2 H), 7.17–7.08 (m, 5 H), 6.90 (d, J = 7.9 Hz, 2 H), 6.69 (t, J = 7.3 Hz, 1 H), 5.98 (dd, J = 8.2, 1.3 Hz, 2 H), 5.64 (dd, J = 5.3, 2.4 Hz, 2 H), 4.26 (dd, J = 5.3, 2.4 Hz, 2 H), 1.33 (s, 18 H); 13C NMR (126 MHz, CDCl3) δ 174.0, 148.8, 146.0, 133.0, 131.2, 130.3, 129.1, 128.7, 128.4, 126.2, 123.9, 123.5, 122.3, 119.6, 116.8, 62.7, 53.1, 34.8, 31.1 ppm; IR (KBr): ν 2952 (m), 2922 (m), 2854 (m), 1710 (m), 1695 (s), 1655 (s), 1435 (m), 1412 (m), 1335 (m), 1235 (m), 1078 (m), 756 (m), 698 (m) cm−1; HRMS (EI) m/z: calcd. for [C36H36N2O2]: 528.2777; found: 528.2780.
N-(2-Ethylhexyl)acenphthylene-5,6-dicarboxylimide adduct 12
A mixture of 8a (103 mg, 0.232 mmol), Et3N (0.1 mL, 0.721 mmol) and N-(2-ethylhexyl)acenphthylene-5,6-dicarboxylimide 11 (112 mg, 0.336 mmol) in dry-acetonitrile (10 mL) was stirred at reflux under N2 for overnight. Concentration in vácuo gave a residue that was subjected to column chromatography on silica gel (elution with chloroform) to afford 12 (50.2 mg, 0.073 mmol) as a yellow solid in 31% yield. M.p. 152−153 °C; 1H NMR (400 MHz, CDCl3) δ 8.14 (dd, J = 7.4, 1.4 Hz, 2 H), 7.30 (dd, J = 7.6, 2.0 Hz, 2 H), 7.26–7.07 (m, 4 H), 7.01–6.97 (m, 4 H), 6.66 (t, J = 7.2 Hz, 1 H), 5.74 (dd, J = 5.4, 2.2 Hz, 2 H), 5.18 (dd, J = 6.0, 1.6 Hz, 2 H), 4.00–3.91 (m, 2 H), 1.83–1.77 (m, 1 H), 1.36–1.20 (m, 26 H), 0.88–0.81 (m, 6 H) ppm; 13C NMR (100 MHz, CDCl3) δ 164.44, 164.40, 150.5, 147.7, 147.3, 138.8, 132.7, 132.6, 132.5, 131.5, 129.0, 125.5, 123.9, 122.6, 122.4, 120.9, 119.38, 119.35, 119.0, 116.7, 63.4, 58.3, 43.9, 37.9, 34.5, 30.9, 30.7, 28.7, 24.1, 23.0, 14.0, 10.6 ppm; IR (KBr): ν 2960 (m), 1699 (s), 1662 (s), 1626 (s), 1598 (m), 1497 (m), 1456 (m), 1419 (m), 1335 (m), 1235 (m), 758 (m), 462 (m), 418 (m) cm−1; UV/Vis (DCM): λmax (log ε) 378 (4.12), 359 (4.24), 345 (4.16), 234 (4.00); FL (DCM): λFL 686 nm (ΦSN < 0.01); MS (CI+, 70 eV) m/z 689 ([M + H]+, 100), 358 (22), 336 (43); HRMS (CI+, 70 eV) Calculated (C48H53N2O2) 689.4107 ([M + H]+), Found: 689.4118.
N-Phenyl-2-azaphenalenyl dimer 13
A Soxhlet extractor was equipped with a reflux condensor and a 50 mL of flask containing with Et3N (5 mL) and hexane (35 mL). The filter paper for the Soxhlet exstractor was charged with 8a (500 mg, 1.1 mmol) and placed in the apparatus, and purged with N2 gas. The flask was heated to bring about reflux for 2 h. Then, brown solids was formed on the filter paper. The brown solid formed on the filter paper was removed by dissolving in cyclohexane. The cyclohexane solution was concentrated in vácuo to give dimer 13 (206 mg, 0.29 mmol) as a colorless solid in 51% yield. M.p. > 300 °C; 1H NMR (500 MHz, CDCl3): δ 7.60 (s, 4 H), 7.60 (s, 2 H), 6.83 (m, 4 H, m-H of Ph), 6.45 (m, 6 H, o,p-H of Ph), 5.06 (s, 4 H), 1.47 (s, 36 H); 13C NMR (126 MHz, CDCl3) δ 150.1, 148.2, 133.4, 132.3, 128.5, 126.9, 122.2, 120.2, 118.5, 116.4, 63.8, 34.9, 31.5 ppm; IR (KBr): ν 2961 (s), 2360 (m), 1633 (m), 1594 (m), 1496 (m), 1397 (m), 1369 (m), 1243 (m), 1060 (m), 757 (m) cm−1; UV/Vis (DCM): λmax (log ε) 260 (4.12), 295 (3.89) nm; HRMS (EI) m/z: calcd. for [C52H58N2]: 710.4600; found: 710.4608.
N-2,6-Di(isopropyl)phenyl-5,8-di-tert-butyl-2-azaphenalenyl 7b
In a glove box filled with N2, a solution of 8b (55 mg, 0.11 mmol) and NaH (97 mg, 4.0 mmol) in THF (4 mL) was stirred at rt for 3 h. Filtration of the mixture gave a filtrate that was concentrated in vácuo to afford 7b as a green solid (46 mg, 0.11 mmol). According to the NMR monitoring (in THF-d8), 7b was generated quantitatively. Decomp. 101−102 °C; 1H NMR (600 MHz, THF-d8): δ 7.38 (t, J = 7.8 Hz, 1 H, H-11), 7.28 (d, J = 7.8 Hz, 2 H, H-10), 6.19 (d, J = 1.8 Hz, 2 H, H-5), 5.67 (d, J = 1.8 Hz, 2 H, H-3), 5.62 (s, 2 H, H-1), 3.55 (sep, J = 7.2 Hz, 2 H, H-13), 1.30 (d, J = 7.2 Hz, 12 H, H-12), 1.15 (s, 18 H, H-14); 13C NMR (150 MHz, THF-d8, rt) δ 151.9 (s, C-4), 144.5 (s, C-9), 141.3 (s, C-8), 140.5 (s, C-6), 138.0 (s, C-2), 136.6 (s, C-7), 130.4 (d, C-11), 125.0 (d, C-10), 118.0 (d, C-1), 112.3 (d, C-5), 103.1 (d, C-3), 34.6 (s, C-15), 31.2 (q, C-14), 28.8 (d, C-13), 25.0 (q, C-12) ppm; IR (KBr): ν 2962 (s), 2867 (m), 1610 (s), 1568 (s), 1459 (m), 1423 (m), 1361 (s), 1332 (m), 1279 (m), 851 (m), 802 (m), 764 (m) cm−1; UV/Vis (DCM): λmax (log ε) 332 (2.87), 423 (3.45), 614 (2.24), 665 (2.34), 727 (2.19) nm; HRMS (EI) m/z: calcd. for [C32H41N]: 439.3239; found: 439.3238.
1H, 11B, 19F and 13C NMR, and Mass spectra of new compounds
See Supplementary Figs. 11–16 and 18–31.
Mass spectra of new compounds
See Supplementary Figs. 32–41.
X-ray diffraction analysis
Single crystals of 7b for X-ray analysis were obtained by slow evaporation from an n-hexane solution. X-ray diffraction data were collected on a Rigaku XtaLAB Synergy-S diffractometer equipped with HyPix-6000HE Hybrid Photon Counting (HPC) X-ray detector with graphite-monochromated MoKα (λ = 0.71073 Å) radiation, and Φ and ω scans at a maximum 2θ value of 61.8. The crystal was kept at 123 K during data collection. Using Olex234, the structure was solved with the ShelXT35 structure solution program using Intrinsic Phasing and refined with the ShelXL36 refinement package using Least Squares minimization.
A single crystal of 13 for X-ray analysis was obtained by slow evaporation from a toluene solution. X-ray diffraction data were collected on a Rigaku Rapid Auto diffractometer with graphite-monochromated MoKα (λ = 0.71075 Å) radiation, and Φ and ω scans at a maximum 2θ value of 50.7°. The structures were solved by a direct method using SHELXL Version 2018/337. All non-hydrogen atoms were refined anisotropically by full-matrix least-squares on F2 using SHELXT version 2018/238. Hydrogen atoms of 13 were refined using the riding model. All calculations were performed using the CrystalStructure crystallographic software package39 except for refinement, which was performed using SHELXL Version 2018/3. Crystallographic details are given in CIF files (Supplementary Data 1 and 2). The detailed crystallographic data for both the compounds are listed in Supplementary Tables 1 and 4.
HOMA calculations
HOMA values of 7b and naphthalene were calculated using C–C and C–N bond lengths derived from the X-ray crystallographic structure, according to the following equation HOMA = 1 – α/nΣ(Ropt – Ri)2, where n is the number of bonds taken in the summation, α is an empirical constant, Ropt is an optimal bond length and Ri is a bond length of ith bond. Ropt.1.388 (C–C) and 1.334 (C–N) Å and α = 257.7 (C–C) and 93.52 (C–N) were used22.
Theoretical calculations
All calculations were conducted using the Gaussian 09 program32. The geometries of 2, 3, 4, 5a‒c, and naphthalene were optimized with the RB3LYP-D3 functional and 6–311 G* basis set. The optimized geometries were used for calculations of the other properties. The values of y of 5a and 5b were obtained from the occupation number of the lowest unoccupied natural orbital (LUNO) (y = nL) at the LC-UBLYP(μ = 0.33 bohr–1)/6–311 G* level. The NICS(1) values of 2, 3, 5a, 5b, and naphthalene were calculated at the GIAO-HF/6–311 + G* level. Electronic excitation properties of 5b were evaluated by using the TD-DFT method RB3LYP and 6–311 + G* basis set.
Data availability
Data for the crystal structures reported in this paper have been deposited at the Cambridge Crystallographic Data Centre (CCDC) under the deposition numbers CCDC 1950740 (7b) and 1950741 (13). Copies of these data can be obtained free of charge via www.ccdc.cam.ac.uk/data_rquest/cif. All other data generated during this study are available from the corresponding author on request.
References
Strecker, A. On a peculiar oxidation by alloxan. Justus Liebigs Ann. Chem. 123, 363–365 (1862).
Coldham, I. & Hufton, R. Intramolecular dipolar cycloaddition reactions of azomethine ylides. Chem. Rev. 105, 2765–2809 (2005).
Pinho e Melo, T. M. V. D. Conjugated Azomethine Ylides. Eur. J. Org. Chem. 2006, 2873–2888 (2006).
Tang, S., Zhang, X., Sun, J., Niu, D. & Chruma, J. J. 2-Azaallyl anions, 2-azaallyl cations, 2-azaallyl radicals, and azomethine ylides. Chem. Rev. 118, 10393–10457 (2018).
Kröhnke, F. Synthesen mit Hilfe von Pyridiniumsalzen. Angew. Chem. 65, 605–627 (1953).
O’Brien, S. & Smith, D. C. C. The synthesis of heterocyclic analogues of phenalene (perinaphthene), containing one hetero-atom. J. Chem. Soc. 2907‒2917 (1963).
Farquhar, D. & Leaver D. Synthesis of pyrido[2,1,6-de]quinolizine (cycl[3,3,3]azine). J. Chem. Soc. D. 1969, 24‒25 (1969).
Sha, C.-K. & Wang, D.-C. Synthesis of the parent compound and N-substituted derivatives of 1H-benz[de]isoquinoline and benzo[de]isoquinolinium-1-ide. Tetrahedron 50, 7495–7502 (1994).
Zheng, S., Lan, J., Khan, S. I. & Rubin, Y. Synthesis, characterization, and coordination chemistry of the 2-azaphenalenyl radical. J. Am. Chem. Soc. 125, 5786–5791 (2003).
Berger, R. et al. Synthesis of nitrogen-doped zigzag-edge peripheries: dibenzo-9a-azaphenalene as repeating unit. Angew. Chem. Int. Ed. 53, 10520–10524 (2014).
Berger, R., Wagner, M., Feng, X. & Müllen, K. Polycyclic aromatic azomethine ylides: a unique entry to extended polycyclic heteroaromatics. Chem. Sci. 6, 436–441 (2015).
Ito, S., Tokimaru, Y. & Nozaki, K. Isoquinolino[4,3,2-de]phenanthridine: synthesis and its use in 1,3-dipolar cycloadditions to form nitrogen-containing polyaromatic hydrocarbons. Chem. Commun. 51, 221–224 (2015).
Tokimaru, Y., Ito, S. & Nozaki, K. A hybrid of corannulene and azacorannulene: synthesis of a highly curved nitrogen-containing buckybowl. Angew. Chem. Int. Ed. 57, 9818–9822 (2018).
Tokimaru, Y., Ito, S. & Nozaki, K. Synthesis of pyrrole-fused corannulenes: 1,3-dipolar cycloaddition of azomethine ylides to Corannulene. Angew. Chem. Int. Ed. 57, 15560–15564 (2018).
Arikawa, S., Shimizu, A. & Shintani, R. Azoniadibenzo[a,j]phenalenide: A polycyclic zwitterion with singlet biradical character. Angew. Chem. Int. Ed. 58, 6415–6419 (2019).
Kawajiri, I. et al. π-Extended fluoranthene imide derivatives: synthesis, structures, and electronic and optical properties. Can. J. Chem. 95, 371–380 (2017).
Yamamoto, Y. et al. Synthesis and properties of a decacyclene monoimide and a naphthalimide derivative as three-dimensional acceptor-donor-acceptor system. Chem. Asian J. 13, 790–798 (2018).
Ishikawa, H., Katayama, K., Nishida, J., Kitamura, C. & Kawase, T. Fluoranthene and its π-extended diimides: construction of new electron acceptors. Tetrahedron Lett. 59, 3782–3786 (2018).
Katayama, K., Kawajiri, I., Okano, Y., Nishida, J. & Kawase, T. Highly polarized benzo[k]fluoranthene imide derivatives: displaying large solvatofluorochromism, dual fluorescence and aggregation induced emission associated with excited-state intramolecular charge transfer. ChemPlusChem 84, 722–729 (2019).
Guerra, P. V. & Yaylayan, V. Dimerization of azomethine ylides: An alternate route to pyrazine formation in the Maillard reaction. J. Agric. Food Chem. 58, 12523–12529 (2010).
Yang, Y. et al. Facile Synthesis of π-extended viologens: electron-deficient polycyclic aza-aromatics. Chem. Eur. J. 23, 7409–7413 (2017).
Krygowski, T. M. Crystallographic studies of inter- and intramolecular interactions reflected in aromatic character of π-electron systems. J. Chem. Inf. Compt. Sci. 33, 70–78 (1993).
Zborowski, K. K., Alkorta, I., Elguero, J. & Proniewicz, L. M. Calculation of the HOMA model parameters for the carbon-boron bond. Struct. Chem. 23, 595–600 (2012).
Cruickshank, D. W. J. A detailed refinement of the crystal and molecular structure of naphthalene. Acta Crystllogr 10, 504–508 (1957).
Chen, Z. et al. Nucleus-independent chemical shifts (NICS) as an aromaticity criterion. Chem. Rev. 105, 3842–3888 (2005).
Sethson, I., Johnels, D., Edlund, U. & Sygula, A. Electronic and ring current structure of phenalenyl Ions. J. Chem. Soc. Perkin Trans. 2, 1339–1341 (1990).
Hempenius, M. A. et al. Spectrometry and reactivity of phenalenyl anions. J. Phys. Org. Chem. 7, 296–302 (1994).
Farquhar, D., Gough, T. T. & Leaver, D. Heterocyclic compounds with bridgehead nitrogen atoms. Part V. Pyrido[2,1,6-de]quinolizines (cycl[3.3.3]azines). J. Chem. Soc. Perkin Trans. 1, 341–355 (1976).
Schröder, H. V. & Schalley, C. A. Tetrathiafulvalene – a redox-switchable building block to control motion in mechanically interlocked molecules. Beilstein J. Org. Chem. 14, 2163–2185 (2018).
Nakasuji, K., Yamaguchi, M. & Murata, I. First realization of threefold fluxionality in polycyclic conjugated hydrocarbon-metal complexes: synthesis and dynamic NMR study of [Pd(η3-phenalenyl)(tmeda)]+PF\(_{6} ^{-}\) and its methyl derivative. J. Am. Chem. Soc. 108, 325–3327 (1986).
Morris, J. V., Mahaney, M. A. & Huber, J. R. Fluorescence quantum yield determinations. 9,10-Diphenylanthracene as a reference standard in different solvents. J. Phys. Chem. 80, 969–974 (1976).
Frisch, M. J. et al. Gaussian 09, Revision D.01. (Gaussian, Inc., Wallingford, CT, 2013).
Altieri, A. et al. Electrochemically switchable hydrogen-bonded molecular shuttles. J. Am. Chem. Soc. 125, 8644–8654 (2003).
Dolomanov, O. V., Bourhis, L. J., Gildea, R. J., Howard, J. A. K. & Puschmann, H. OLEX2: a complete structure solution, refinement and analysis program. J. Appl. Crystallogr 42, 339–341 (2009).
Sheldrick, G. M. SHELXT – Integrated space-group and crystal-structure determination. Acta Crystallogr. Sect. A Found. Adv. 71, 3–8 (2015).
Sheldrick, G. M. Crystal structure refinement with SHELXL. Acta Crystallogr. Sect. C. Struct. Chem. 71, 3–8 (2015).
Sheldrick, G. M. A short history of SHELX. Acta Crystallogr. Sect. A Found. Crystallogr. 64, 112–122 (2008).
Sheldrick, G. M. Integrating space group determination and structure solution. Acta Cryst. A70, C1437 (2014).
CrystalStructure 4.3: Crystal Structure Analysis Package, Rigaku Corporation (2000–2018). Tokyo 196-8666, Japan.
Acknowledgements
We thank Dr. Hiroyasu Sato (Rigaku Corporation) for assistance with the X-ray analysis of the dimer 13. This study was financially supported by Hyogo prefecture and a JSPS Grant-in-Aid for Scientific Research (C) (JP16K05896, JP18K05091).
Author information
Authors and Affiliations
Contributions
T.K. conceived the project, designed molecules, interpreted the results and drafted the paper. K.K. synthesized all compounds, conducted the UV/vis absorption, fluorescence, IR spectroscopy and Cyclic Voltammetry studies, and carried out detailed DFT and TD-DFT calculations, under the supervision of T.K. J.N. assisted K.K. C.K. determined the crystal structure of 13. A.K. determined the crystal structure of 7b, performed mass spectrometric analysis of all compounds, and conducted detailed DFT and TD-DFT calculations on 2, 4, 5a‒c, 7a and 7b. K.H. performed NMR measurements and chemical shift assignments of 7b and 12, and performed 11B- and 19F-NMR measurements on 8a and 8b. A.K. and K.H. were supervised by M.Y.
Corresponding author
Ethics declarations
Competing interests
The authors declare no competing interests.
Additional information
Publisher’s note Springer Nature remains neutral with regard to jurisdictional claims in published maps and institutional affiliations.
Supplementary information
Rights and permissions
Open Access This article is licensed under a Creative Commons Attribution 4.0 International License, which permits use, sharing, adaptation, distribution and reproduction in any medium or format, as long as you give appropriate credit to the original author(s) and the source, provide a link to the Creative Commons license, and indicate if changes were made. The images or other third party material in this article are included in the article’s Creative Commons license, unless indicated otherwise in a credit line to the material. If material is not included in the article’s Creative Commons license and your intended use is not permitted by statutory regulation or exceeds the permitted use, you will need to obtain permission directly from the copyright holder. To view a copy of this license, visit http://creativecommons.org/licenses/by/4.0/.
About this article
Cite this article
Katayama, K., Konishi, A., Horii, K. et al. Isolation and characterisation of a stable 2-azaphenalenyl azomethine ylide. Commun Chem 2, 136 (2019). https://doi.org/10.1038/s42004-019-0236-y
Received:
Accepted:
Published:
DOI: https://doi.org/10.1038/s42004-019-0236-y
Comments
By submitting a comment you agree to abide by our Terms and Community Guidelines. If you find something abusive or that does not comply with our terms or guidelines please flag it as inappropriate.