Abstract
Metformin has attracted increasing interest for its potential benefits in extending healthspan and longevity. This study examined the effects of early-life metformin treatment on the development and metabolism of C57BL/6 J (B6) mice, with metformin administered to juvenile mice from 15 to 56 days of age. Metformin treatment led to decreased body weight in both sexes (P < 0.05, t-test). At 9 weeks of age, mice were euthanized and organ weights were recorded. The relative weight of retroperitoneal fat was decreased in females, while relative weights of perigonadal and retroperitoneal fat were decreased, and relative liver weight was increased in males (P < 0.05, t-test). Glucose and insulin tolerance tests (GTT and ITT) were conducted at the age of 7 weeks. ANOVA revealed a significant impairment in insulin sensitivity by the treatment, and a significantly interactive effect on glucose tolerance between sex and treatment, underscoring a disparity in GTT between sexes in response to the treatment. Metformin treatment reduced circulating insulin levels in fasting and non-fasting conditions for male mice, with no significant alterations observed in female mice. qRT-PCR analysis of glucose metabolism-related genes (Akt2, Glut2, Glut4, Irs1, Nrip1, Pi3k, Pi3kca, Pkca) in the liver and skeletal muscle reveals metformin-induced sex- and organ-specific effects on gene expression. Comparison with previous studies in heterogeneous UM-HET3 mice receiving the same treatment suggests that genetic differences may contribute to variability in the effects of metformin treatment on development and metabolism. These findings indicate that early-life metformin treatment affects development and metabolism in both sex- and genetics-dependent manners.







Similar content being viewed by others
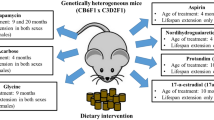
References
Lv ZQ, Guo YJ. Metformin and its benefits for various diseases. Front Endocrinol. 2020;11:191.
Rena G, Hardie DG, Pearson ER. The mechanisms of action of metformin. Diabetologia. 2017;60(9):1577–85.
Rojas LB, Gomes MB. Metformin: an old but still the best treatment for type 2 diabetes. Diabetol Metab Syndr. 2013;5(1):6.
Scapaticci S, et al. Non-alcoholic fatty liver disease in obese youth with insulin resistance and type 2 diabetes. Front Endocrinol. 2021;12:639548.
Arshad T, et al. Nonalcoholic fatty liver disease prevalence trends among adolescents and young adults in the United States, 2007–2016. Hepatol Commun. 2021;5(10):1676–88.
Rowan JA, et al. Metformin in gestational diabetes: the offspring follow-up (MiG TOFU): body composition at 2 years of age. Diabetes Care. 2011;34(10):2279–84.
Ro TB, et al. Growth, body composition and metabolic profile of 8-year-old children exposed to metformin in utero. Scand J Clin Lab Invest. 2012;72(7):570–5.
Rowan JA, et al. Metformin in gestational diabetes: the offspring follow-up (MiG TOFU): body composition and metabolic outcomes at 7–9 years of age. Bmj Open Diabetes Res Care. 2018;6(1):e000456.
Ibanez L, et al. Metformin treatment for four years to reduce total and visceral fat in low birth weight girls with precocious pubarche. J Clin Endocrinol Metab. 2008;93(5):1841–5.
Diaz M, et al. Circulating GDF15 concentrations in girls with low birth weight: effects of prolonged metformin treatment. Pediatr Res. 2023;93(4):964–68.
Ibanez L, et al. Early metformin therapy to delay menarche and augment height in girls with precocious pubarche. Fertil Steril. 2011;95(2):727–30.
Chen LG, et al. OCT1 is a high-capacity thiamine transporter that regulates hepatic steatosis and is a target of metformin. Proc Natl Acad Sci USA. 2014;111(27):9983–8.
Pawlyk AC, et al. Metformin pharmacogenomics: current status and future directions. Diabetes. 2014;63(8):2590–9.
Diaz M, et al. Responsiveness to metformin in girls with androgen excess: collective influence of genetic polymorphisms. Fertil Steril. 2011;96(1):208-U637.
Zhu Y, et al. Metformin treatment of juvenile mice alters aging-related developmental and metabolic phenotypes. Mech Ageing Dev. 2022;201:111597.
Martin-Montalvo A, et al. Metformin improves healthspan and lifespan in mice. Nat Commun. 2013;4:2192.
Kulkarni AS, Gubbi S, Barzilai N. Benefits of metformin in attenuating the hallmarks of aging. Cell Metab. 2020;32(1):15–30.
Anisimov VN, et al. If started early in life, metformin treatment increases life span and postpones tumors in female SHR mice. Aging (Albany NY). 2011;3(2):148–57.
Panici JA, et al. Early life growth hormone treatment shortens longevity and decreases cellular stress resistance in long-lived mutant mice. FASEB J. 2010;24(12):5073–9.
Li X, et al. Transient early life growth hormone exposure permanently alters brain, muscle, liver, macrophage, and adipocyte status in long-lived Ames dwarf mice. FASEB J. 2022;36(7):e22394.
Drake JC, et al. Long-lived crowded-litter mice have an age-dependent increase in protein synthesis to DNA synthesis ratio and mTORC1 substrate phosphorylation. Am J Physiol Endocrinol Metab. 2014;307(9):E813–21.
Kurup K, et al. Litter expansion alters metabolic homeostasis in a sex specific manner. PLoS ONE. 2021;16(9):e0237199.
Shindyapina AV, et al. Rapamycin treatment during development extends life span and health span of male mice and Daphnia magna. Sci Adv. 2022;8(37):eabo5482.
Aiello G, et al. Transient rapamycin treatment during developmental stage extends lifespan in Mus musculus and Drosophila melanogaster. EMBO Rep. 2022;23(9):e55299.
Yuan R, et al. Aging in inbred strains of mice: study design and interim report on median lifespans and circulating IGF1 levels. Aging Cell. 2009;8(3):277–87.
Yuan R, et al. Genetic coregulation of age of female sexual maturation and lifespan through circulating IGF1 among inbred mouse strains. Proc Natl Acad Sci U S A. 2012;109(21):8224–9.
LaMoia TE, Shulman GI. Cellular and molecular mechanisms of metformin action. Endocr Rev. 2021;42(1):77–96.
Iversen AB, et al. Results from C-11-metformin-PET scans, tissue analysis and cellular drug-sensitivity assays questions the view that biguanides affects tumor respiration directly. Sci Rep. 2017;7(1):9436.
Nwabueze OP, et al. Comparative studies of palmatine with metformin and glimepiride on the modulation of insulin dependent signaling pathway in vitro, in vivo & ex vivo. Pharmaceuticals (Basel). 2022;15(11):1317.
Dowling RJO, et al. Metformin inhibits mammalian target of rapamycin-dependent translation initiation in breast cancer cells. Can Res. 2007;67(22):10804–12.
Zhou GC, et al. Role of AMP-activated protein kinase in mechanism of metformin action. J Clin Investig. 2001;108(8):1167–74.
Owen MR, Doran E, Halestrap AP. Evidence that metformin exerts its anti-diabetic effects through inhibition of complex 1 of the mitochondrial respiratory chain. Biochem J. 2000;348:607–14.
Wiernsperger NF, Bailey CJ. The antihyperglycaemic effect of metformin - therapeutic and cellular mechanisms. Drugs. 1999;58:31–9.
Bartelt A, Heeren J. Adipose tissue browning and metabolic health. Nat Rev Endocrinol. 2014;10(1):24–36.
Hardie DG. Sensing of energy and nutrients by AMP-activated protein kinase. Am J Clin Nutr. 2011;93(4):891s–6s.
Guo WR, et al. Metformin alleviates steatohepatitis in diet-induced obese mice in a SIRT1-dependent way. Front Pharmacol. 2021;12:704112.
Ji S, Wang L, Li L. Effect of metformin on short-term high-fat diet-induced weight gain and anxiety-like behavior and the gut microbiota. Front Endocrinol (Lausanne). 2019;10:704.
Kim EK, et al. Metformin prevents fatty liver and improves balance of white/brown adipose in an obesity mouse model by inducing FGF21. Mediators Inflamm. 2016;2016:5813030.
Schoonejans JM, et al. Maternal metformin intervention during obese glucose-intolerant pregnancy affects adiposity in young adult mouse offspring in a sex-specific manner. Int J Mol Sci. 2021;22(15):8104.
McNulty SJ, et al. A randomized trial of sibutramine in the management of obese type 2 diabetic patients treated with metformin. Diabetes Care. 2003;26(1):125–31.
Dayabandara M, et al. Antipsychotic-associated weight gain: management strategies and impact on treatment adherence. Neuropsychiatr Dis Treat. 2017;13:2231–41.
Alberti KG, Zimmet P, Shaw J. International Diabetes Federation: a consensus on type 2 diabetes prevention. Diabet Med. 2007;24(5):451–63.
Artinian NT, et al. Interventions to promote physical activity and dietary lifestyle changes for cardiovascular risk factor reduction in adults a scientific statement from the American Heart Association. Circulation. 2010;122(4):406–41.
Anisimov VN, et al. Sex differences in aging, life span and spontaneous tumorigenesis in 129/Sv mice neonatally exposed to metformin. Cell Cycle. 2015;14(1):46–55.
Tartarin P, et al. Metformin exposure affects human and mouse fetal testicular cells. Hum Reprod. 2012;27(11):3304–14.
Coqueret O. New roles for p21 and p27 cell-cycle inhibitors: a function for each cell compartment? Trends Cell Biol. 2003;13(2):65–70.
Nguyen TM. Impact of 5’-amp-activated protein kinase on male Gonad and Spermatozoa functions. Front Cell Dev Biol. 2017;5:25.
Riera MF, et al. Signal transduction pathways in FSH regulation of rat Sertoli cell proliferation. Am J Physiol-Endocrinol Metab. 2012;302(8):E914–23.
Rindone GM, et al. Metformin counteracts the effects of FSH on rat Sertoli cell proliferation. Reproduction. 2018;156(2):93–101.
Faure M, et al. Metformin in reproductive biology. Front Endocrinol. 2018;9:675.
Sun SMS, et al. National estimates of the timing of sexual maturation and racial differences among US children. Pediatrics. 2002;110(5):911–9.
Rahmani J, et al. The influence of fasting and energy restricting diets on IGF-1 levels in humans: a systematic review and meta-analysis. Ageing Res Rev. 2019;53:100910.
Cheng CW, et al. Prolonged fasting reduces IGF-1/PKA to promote hematopoietic-stem-cell-based regeneration and reverse immunosuppression. Cell Stem Cell. 2014;14(6):810–23.
Nencioni A, et al. Fasting and cancer: molecular mechanisms and clinical application. Nat Rev Cancer. 2018;18(11):707–19.
Cai D, et al. Insulin-like growth factor 1/mammalian target of rapamycin and AMP-activated protein kinase signaling involved in the effects of metformin in the human endometrial cancer. Int J Gynecol Cancer. 2016;26(9):1667–72.
Hamed HO, et al. Metformin versus laparoscopic ovarian drilling in clomiphene- and insulin-resistant women with polycystic ovary syndrome. Int J Gynaecol Obstet. 2010;108(2):143–7.
Yates MS, et al. Prospective randomized biomarker study of metformin and lifestyle intervention for prevention in obese women at increased risk for endometrial cancer. Cancer Prev Res (Phila). 2018;11(8):477–90.
Yang X, et al. The influence of metformin on IGF-1 levels in humans: a systematic review and meta-analysis. Pharmacol Res. 2020;151:104588.
Vitale G, et al. ROLE of IGF-1 system in the modulation of longevity: controversies and new insights from a centenarians’ perspective. Front Endocrinol (Lausanne). 2019;10:27.
Berryman DE, et al. Role of the GH/IGF-1 axis in lifespan and healthspan: lessons from animal models. Growth Horm IGF Res. 2008;18(6):455–71.
Yuan R, et al. Genetic differences and longevity-related phenotypes influence lifespan and lifespan variation in a sex-specific manner in mice. Aging Cell. 2020;19(11):e13263.
Guido M, et al. Effect of metformin on the growth hormone response to growth hormone-releasing hormone in obese women with polycystic ovary syndrome. Fertil Steril. 2005;84(5):1470–6.
Zhou K, et al. Heritability of variation in glycaemic response to metformin: a genome-wide complex trait analysis. Lancet Diabetes Endocrinol. 2014;2(6):481–7.
Rutherford SL. From genotype to phenotype: buffering mechanisms and the storage of genetic information. BioEssays. 2000;22(12):1095–105.
Freemark M, Bursey D. The effects of metformin on body mass index and glucose tolerance in obese adolescents with fasting hyperinsulinemia and a family history of type 2 diabetes. Pediatrics. 2001;107(4):E55.
Herman R, et al. Metformin and insulin resistance: a review of the underlying mechanisms behind changes in GLUT4-mediated glucose transport. Int J Mol Sci. 2022;23(3):1264.
McCreight LJ, et al. Metformin increases fasting glucose clearance and endogenous glucose production in non-diabetic individuals. Diabetologia. 2020;63(2):444–7.
Gormsen LC, et al. Metformin increases endogenous glucose production in non-diabetic individuals and individuals with recent-onset type 2 diabetes. Diabetologia. 2019;62(7):1251–6.
Christensen MM, et al. Endogenous glucose production increases in response to metformin treatment in the glycogen-depleted state in humans: a randomised trial. Diabetologia. 2015;58(11):2494–502.
Bailey CJ, Wilcock C, Scarpello JH. Metformin and the intestine. Diabetologia. 2008;51(8):1552–3.
Davidson MB. Metformin should not be used to treat prediabetes. Diabetes Care. 2020;43(9):1983–7.
Schoonejans JM, et al. Sex-specific effects of maternal metformin intervention during glucose-intolerant obese pregnancy on body composition and metabolic health in aged mouse offspring. Diabetologia. 2022;65(12):2132–45.
Faulkner JL, BelindeChantemele EJ. Sex hormones, aging and cardiometabolic syndrome. Biol Sex Differ. 2019;10(1):30.
Ilias I, Rizzo M, Zabuliene L. Metformin: sex/gender differences in its uses and effects-narrative review. Medicina (Kaunas). 2022;58(3):430.
Rosell M, Jones MC, Parker MG. Role of nuclear receptor corepressor RIP140 in metabolic syndrome. Biochim Biophys Acta. 2011;1812(8):919–28.
White R, et al. Role of RIP140 in metabolic tissues: connections to disease. FEBS Lett. 2008;582(1):39–45.
Wang J, et al. Deletion of Nrip1 extends female mice longevity, increases autophagy, and delays cell senescence. J Gerontol A Biol Sci Med Sci. 2018;73(7):882–92.
Fritah A, et al. Absence of RIP140 reveals a pathway regulating glut4-dependent glucose uptake in oxidative skeletal muscle through UCP1-mediated activation of AMPK. PLoS ONE. 2012;7(2):e32520.
Kiskinis E, et al. RIP140 directs histone and DNA methylation to silence Ucp1 expression in white adipocytes. EMBO J. 2007;26(23):4831–40.
Funding
This study was supported by grants from the William E. McElroy Charitable Foundation, the National Institute of Aging (R21AG062985), the American Diabetes Association (1–19-IBS-126) to A.B., the School of Medicine at Southern Illinois University Research Seed Grant to R.Y., the Southern Illinois University System Collaborative Grant to R.Y. and B.E., and 2022 AOA Student Research Fellowship to M.E.
Author information
Authors and Affiliations
Contributions
All authors contributed to the study conception and design. Material preparation, data collection, and analysis were performed by Y.Z., M.E., D.M., X.H., P.D., and R.Y. The first draft of the manuscript was written by Y.Z., M.E., and R.Y., all authors commented on previous versions of the manuscript. All authors read and approved the final manuscript.
Corresponding author
Ethics declarations
Conflict of interest
The authors declare no competing interests.
Additional information
Publisher's Note
Springer Nature remains neutral with regard to jurisdictional claims in published maps and institutional affiliations.
Supplementary Information
Below is the link to the electronic supplementary material.
About this article
Cite this article
Zhu, Y., Engmann, M., Medina, D. et al. Metformin treatment of juvenile mice alters aging-related developmental and metabolic phenotypes in sex-dependent and sex-independent manners. GeroScience 46, 3197–3218 (2024). https://doi.org/10.1007/s11357-024-01067-6
Received:
Accepted:
Published:
Issue Date:
DOI: https://doi.org/10.1007/s11357-024-01067-6