Applications of Bioadhesives: A Mini Review
- 1Department of Biophysics, School of Basic Medical Sciences, Health Science Center, Institute of Medical Engineering, Xi’an Jiaotong University, Xi’an, China
- 2The First Medical Center of Chinese PLA General Hospital, Beijing, China
Bioadhesives have demonstrated their superiority in clinical applications as tissue adhesives, hemostats, and tissue sealants. Because of the intrinsic stickiness, the applications have been expanded to various areas, such as functional wound dressing, factor delivery vehicles, and even medical device fixation. While many literature works discussed the mechanism of bioadhesives, few of them specifically summarized the applications of bioadhesives. To fill in the blanks, this review covers recent research articles and focuses precisely on the applications of bioadhesives which can be generally classified as follows: 1) wound closure, 2) sealing leakage, and 3) immobilization, including those already in the clinic and those showing great potential in the clinic. It is expected that this article will provide a whole picture on bioadhesives’ applications and lead to innovations in the application of bioadhesives in new fields.
Introduction
Bioadhesives have been changing the surgical process with increasing importance and rapid development over the past 30 years (Ge and Chen, 2020; Taboada et al., 2020). The growing interest in producing adhesives and sealants makes them constitute a market share of $38 billion currently (Spotnitz and Burks, 2012; Qu et al., 2018; Liang et al., 2019). Compared with traditional invasive wound closure methods, including sutures, wires, and staples, bioadhesives have less possibility to damage the tissues and can promote wound healing through different mechanisms. For example, the bioadhesives possess antibacterial, anti-inflammatory, and antioxidant properties (Giano et al., 2014; Zhao et al., 2020). Other properties like self-healing and injectability significantly increase bioadhesives’ ease of use (Sun et al., 2020). Preventing leakage is also an essential role of bioadhesives. Leakage happens easily after the surgical process, which is up to 30% in some challenging situations. The leakage will easily lead to pain, inflammation, infection, and a high mortality rate (Artzi, 2013; Slieker et al., 2013; Pausch et al., 2020). With an aim to prevent those postoperative leakages, different bioadhesives have been developed accordingly. FocalSeal® was developed to avoid air leakage during lung surgery. DuraSeal® was designed for the spine and dura sealing. Coseal® was used as an adjunct of suture to prevent the leakage of blood vessels.
Moreover, they can remain stable on the site of application because of the intrinsic adhesion property. So, another important function of bioadhesives is immobilization. They can immobilize themselves as functional wound dressings to promote wound healing without other fixation methods (Yang et al., 2021). They can also be employed as vehicles to deliver functional items like drugs or cells to realize local delivery (Patel et al., 2014; Hu et al., 2021). With the development of smart biomedical devices, like wearable devices, implantable detectors, or sensors, a question has been raised about how to fix those devices on/in the body through noninvasive methods without damaging the tissues or the medical devices, to which the bioadhesive is also a good solution (Hwang et al., 2018; Deng et al., 2021).
Since bioadhesives are being explored in all sorts of fields, there is a need to summarize these applications, including the existing ones and potential ones. However, till now, most reviews focused on either bioadhesives’ adhesion mechanism or their applications on wound closure and leakage prevention; they seldom specifically discussed the overall applications of bioadhesives (Zhu et al., 2018; Bao et al., 2020; Ge and Chen, 2020; Taboada et al., 2020). Hence, in this review, the applications of bioadhesives have been summarized and grouped into three categories (Figure 1): 1) wound closure, 2) sealing leakage, and 3) immobilization. The examples of each category were demonstrated with the hope of providing a whole picture of the applications of bioadhesives and accelerating the innovation of bioadhesives in new fields. It is worthy to note that some bioadhesives own properties of two or three categories. Here, the bioadhesives are grouped according to their primary functions and the authors’ understanding of the bioadhesives.
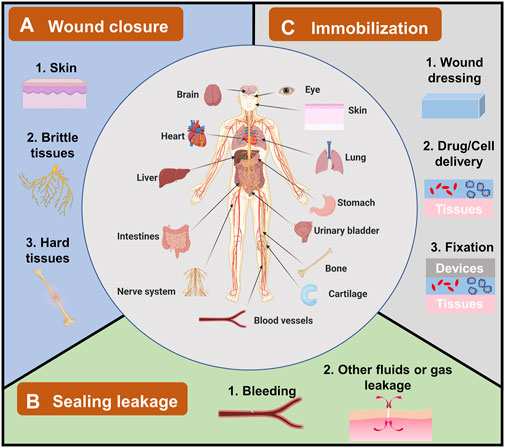
FIGURE 1. The applications of bioadhesives in human bodies and their categories. Bioadhesives have been explored in human bodies in different organs, including the brain, eyes, heart, liver, and skin. Their applications can be grouped into three categories. (A) Wound closure, which has been used in topical skin, and brittle/hard tissues. (B) Sealing leakage, including most explored blood leakage and other fluids or gas leakage. (C) Immobilization for wound dressing, drug/cell delivery, and fixation of devices.
Wound Closure
Wound closure is one of the most widely used applications of bioadhesives (Table 1). Sutures, wires, and staples have been the routine practice of wound closure for many years (Mehdizadeh and Yang, 2013). However, concerns about the scar tissues, secondary injury, foreign body reaction, wicking-induced infection, impaired wound healing process, and complex postoperative care are still waiting to be addressed (Harsha and Vasudha, 2018). As a good alternative, bioadhesives can adhere two wounds together through a noninvasive behavior. Typically, bioadhesives close the wounds by three methods: bringing the two sides of an injury together from the wound surface (Figure 2A), bringing the tissues beneath the surface together (Figure 2B), or closing wounds in both ways (Figure 2C). Firm adhesion is the property needed for all the three types. Moreover, the bioadhesives applied to wounds (Figures 2A,C) should be biodegradable and biocompatible and should not hinder the healing process (Li et al., 2020). The bioadhesives used on the surface are generally tape-like ones with strong cohesion strength (Bae et al., 2013; Yang et al., 2013). Cohesion, which is defined as the internal strength of an adhesive, together with adhesion creates a strong bond; few people conducted in-depth research on cohesion strength alone. However, it is reported that the photo-crosslinking strategy is commonly used to develop tape-like bioadhesives with high cohesion strength. Besides, the double network strategy has also been used to develop bioadhesive tapes with good wound closure efficacy by increasing the cohesion strength (Liu et al., 2018; Yuk et al., 2019; Pausch et al., 2020). Cyanoacrylate-based bioadhesives are the most widely used tissue adhesives for wound closure in the market, initially synthesized in 1949 (Harsha and Vasudha, 2018). Although they are not tape-like, they play roles mainly according to the second type because of their strong adhesion strength. However, their applications on wet tissues were limited because of their water-initiated curing. They also raise security concerns for exothermic polymerization, cytotoxic degradation products, and long degradation time (Bu et al., 2017; Harsha and Vasudha, 2018). So, special attention should be paid to avoid pushing cyanoacrylate-based bioadhesives into the wound, which can cause irritation and foreign body reaction. There are indications of holding wound edges together for at least 30 s before releasing. Because of the brittle property of the barriers formed by cyanoacrylate, it is suggested that cyanoacrylates are not suitable for wounds over joints, like the knees, groins, or hands, where adhesion easily fails because of the skin torsion (Harsha and Vasudha., 2018).
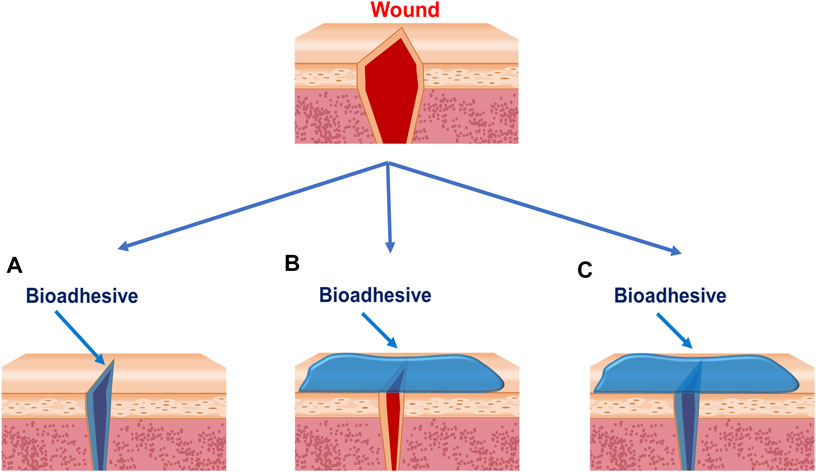
FIGURE 2. Strategies bioadhesives used to close wounds. (A) Bioadhesives are applied between wound edges. (B) Bioadhesives are applied outside of the wounds. (C) Bioadhesives are applied between and outside the wounds.
Skin Closure
Skin closure is one of the main goals for wound closure-targeting bioadhesives which is in high demand because of the increasing workload of general surgery (Lu et al., 2020). This application has expanded popularity also because people pay more attention to their physical appearance. Dermal surgeons prefer using bioadhesives to improve their work efficiency. Patients tend to use noninvasive methods because there is usually less pain and a better cosmetic outcome (Ge and Chen, 2020). Luo et al. developed a new bioadhesive from the skin secretion of Chinese giant salamander. Later, the ability to close the wound was tested on the back of the rats with four incisions (2 cm). At the 5th day of postoperation, the bioadhesive-treated group showed the best healing effect among all groups, with no scar formation, infection, and inflammation (Deng et al., 2019). Du et al. fabricated an adhesive patch with poly-(ethylene glycol) diacrylate/quaternized chitosan/tannic acid based on mussel-inspired chemistry. The efficacy of the wound closure was tested on a full-thickness incision model. It was proved that at day 7 postsurgery, the patch-closed skin incisions exhibited more complete epidermis and dermis structures, and higher collagen deposition levels than the untreated tissues (Du et al., 2019).
Wound Closure of Hard or Brittle Tissues
Other kinds of wound closure, in which bioadhesives show super advantages, are closing wounds of hard and extremely brittle tissues. In hard tissues like bones, bioadhesives provide a quick and straightforward method to fix the broken pieces, especially for small bone fragments (Farrar, 2012). For example, comminuted bone fracture is a severe orthopedic condition. The difficulty in fixation of the small bone pieces often leads to bone reduction, further resulting in bone displacement, bone union deformation, and nonunion. Based on citrate, Xie et al. developed an injectable bioadhesive to fix small bone pieces in comminuted bone fractures (Xie et al., 2015). Hydroxyapatite was added to the system to improve the healing efficacy. It was demonstrated that the bioadhesive increased bone formation with markedly enhanced three-point bending strength compared with the negative control. In extremely brittle or sensitive tissues like nerves, traditional sutures can cause irreversible damage. Besides, skilled surgeons are required for suturing those tissues, which entails prolonged surgical time and surgical skills. In our previous work, the octa-PEG–based bioadhesives have been used to close the nerve transection. After adding lithium chloride, the adhesive-reconnected nerves showed a low level of fibrosis, inflammation, and myoatrophy, as well as robust axonal regeneration and functional recovery (Bu et al., 2020). Corneal is another brittle tissue in which closure can be achieved by the bioadhesives. Shirzaei Sani et al. had engineered a gelatin-based adhesive biomaterial GelCORE to close the eye incision in an ex vivo model. It was found that the mean leak pressure of glue was more significant than that of commercial control groups (Shirzaei Sani et al., 2019).
Sealing Leakage
Leakage is a common complication of surgeries and injuries. After lung resections, the incidence of air leakage was reported to be around 50% (Mueller and Marzluf, 2014). Cerebrospinal fluid leakage, caused by injuries or brain and sinus surgery, can lead to headaches, meningitis, and seizures. Gastric fluid leakage can cause severe tissue damage and infection, which happens easily after surgical procedures. So leakage prevention is vital in reducing operative risks, and decreasing the complications and the cost. Bioadhesives for leakage prevention are also called tissue sealants, which attracted the attention of researchers and have shown great potential in the clinic (Ryu et al., 2011; Nie et al., 2013; Behrens et al., 2014; Chan Choi et al., 2014; Kim et al., 2015; Chen et al., 2017; Yan et al., 2018; Kim et al., 2020). Some examples of the tissue sealants are summarized in table 2.
Bleeding
In this review, bleeding is considered as the leakage of the blood, resulting from trauma, surgical process, diseases, and even some medicines. It is one of the most frequent complications in patients. There are many sealants available in the market for hemostasis, such as Tisseel® (Fibrin sealant), Coseal® (PEG sealant), and Bioglue® (Albumin and Glutaraldehyde). However, they have separate limits. In their indications, Tisseel® is not suggested for massive bleeding; Coseal® and Bioglue® are suggested to be used as adjunctions to sutures or staples. So, sealants with high efficacy are still highly desired for uncontrollable or massive bleeding. Different strategies have been used to develop bioadhesives for hemostasis. Cui et al. developed a hyperbranched polymer sealant with a hydrophobic backbone and hydrophilic adhesive catechol side. By introducing long alkylamine chain into the structure, their sealant showed efficient hemostasis in the rat’s femoral artery bleeding and liver bleeding model (Cui et al., 2019). In our previous work, the concept of fabricating sealants with strong cohesion strength has been used (Bu et al., 2016; Bu et al., 2019). Tough sealants based on ammonolysis-based Tetra-PEG hydrogels were fabricated, which showed promising efficacy in pigskin massive bleeding and rabbit femoral artery section models (Bu et al., 2016). Hemostasis is another critical situation for patients with coagulation disorders, such as hemophilia, Von Willebrand disease, and aged patients taking anticoagulation drugs. Shin et al. presented a hemostatic hypodermic needle that will be able to prevent bleeding following tissue puncture. The surface of the needle was coated with catechol-functionalized chitosan that would be transformed from the solid to the gel phase in situ to seal punctured tissues (Shin et al., 2016). Later, Kim et al. used the catechol-conjugated chitosan to fabricate a hemostatic sponge (Kim et al., 2021). They used preclinical models to evaluate the hemostatic efficacy, including the heparinized rabbit model of femoral artery bleeding, the pig model of traumatic blunt liver injury with hemodilutional and hypothermic coagulopathy, and the anticoagulant-treated rabbit model of liver resection bleeding. A further clinical study performed on 15 patients showed that this sponge demonstrated an excellent hemostatic effect compared with the commercialized controls.
Other Leakages
Except for blood leakage, there are also some other leakage types. In lung surgery, prolonged air leakage is the most common complication after surgical dissection and resection. The criteria of an ideal sealant for lung leakage include the following: 1. The sealant can stand higher burst pressure than that generated during physiological breathing; 2. the sealant should be elastic with a proper elastic modulus to support the inflation and deflation of lung tissue. Annabi et al. used methacryloyl-substituted tropoelastin (MeTro) to engineer a highly flexible sealant (Assmann et al., 2017). After applying MeTro to a porcine model, it was found that the sealant completely sealed the severely leaking lung tissue in the absence of sutures or staples. Urinary fistulas have been considered a severe socioeconomic problem, which occurs most commonly as a result of prolonged obstructed labor, which causes pelvic floor ischemia and, at times, substantial tissue loss (Margules and Rovner, 2019). Kim et al. developed water-immiscible mussel protein–based bioadhesive, which successfully sealed ex vivo urinary fistulas and provided good durability and high compliance (Kim et al., 2015). Liu et al. developed gelatin methacrylate–based double-network hydrogel to manage the leakage of gastric contents without sutures successfully (Liu et al., 2018).
Immobilization
The last category for bioadhesives includes those for immobilization (Table 3). Because of the intrinsic adhesion property, they can immobilize themselves as functional wound dressing or delivery vehicles. By adhering items together, they even can fix other medical devices.
Functional Wound Dressings
Advanced fixation methods are still in need because traditional wound dressing methods lack the ability of adhesion to wounds, which increases the operative difficulty index for both the patients and doctors. Compared with these methods, bioadhesives can easily fix themselves on the wound area, contributing to the increasing popularity of bioadhesives to be used as a functional wound dressing (Liang et al., 2019; Zhao et al., 2017; Blacklow et al., 2019; Han et al., 2017; Han et al., 2017; Gan et al., 2019). They are favorite candidates for skin damage, one of the most common physical injuries in human history. Based on quaternized chitosan (QCS) and benzaldehyde-terminated Pluronic®F127, Qu et al. developed antibacterial bioadhesives with rapid self-healing, extensibility, and compressibility for joints and skin wound healing (Qu et al., 2018). They loaded curcumin into the bioadhesive and found that it significantly accelerated wound healing with a higher granulation tissue thickness in a full-thickness skin defect model. Inspired by embryonic wound closure, Blacklow et al. fabricated mechanically active dressings to accelerate wound healing (Blacklow et al., 2019). The bioadhesive dressing will contract at body temperature, which further applies force to draw the wound edges together in a purse-string–like manner. Adhesive dressings are beneficial in places where the fixation is difficult, like brittle tissues. Lin et al. developed a viscoelastic adhesive patch that accommodates the cyclic deformation of the myocardium. It was found that the patch outperformed most existing acellular epicardial patches in reversing left ventricular remodeling and restoring heart function after both acute and subacute myocardial infarctions in rats (Lin et al., 2019). In addition to the heart, defects from the corneal, cartilage, and calvarial were explored to be treated with bioadhesives with good outcomes (Feng et al., 2016; Han et al., 2018; Lin et al., 2019; Shirzaei Sani et al., 2019).
Delivery Systems
Compared with the traditional hydrogel delivery system, the advantage of bioadhesives in delivery is that they can fix delivered items on the site. Mucoadhesion is very useful in increasing the bioavailability of poorly absorbed drugs by prolonging the residence time in the gastrointestinal tract, leading to reduced dose and dosing frequency (Han et al., 2012; Gong et al., 2017). A lot of mucoadhesive-based delivery systems were developed with some well-summarized reviews (Reddy et al., 2011; Zhang et al., 2016; Zhang et al., 2020; Pathak and Malviya, 2020). Hu et al. encapsulated camptothecin into poly(lactic acid)-hyperbranched polyglycerol-based nano-bioadhesive particles (NPs). Because of the strong bonding of these NPs to squamous cell carcinoma tumor cells, the system significantly reduced the tumor burden and enhanced survival (Hu et al., 2021). Except for the nano/micro scale mucoadhesion, macro-bioadhesives have also been developed to load drugs to achieve better healing efficacy (Zhang et al., 2019; Bu et al., 2020). Cells can also be loaded into the bioadhesives. The use of an appropriate scaffold biomaterial as a cell delivery vehicle can provide a suitable microenvironment to prolong cell viability and present essential factors to direct cell differentiation toward the desired lineages (Khademhosseini and Langer, 2016). Currently, however, a major drawback of the reported cell-laden hydrogels is the weak adhesion to the host tissue at the defective site. Hasani-Sadrabadi et al. used alginate-based photo-crosslinkable bioadhesives to load mesenchymal stem cells. It was found that the cell-loaded adhesive system leads to complete bone regeneration around the ailing dental implants with peri-implant bone loss (Hasani-Sadrabadi et al., 2020).
Fixation of Other Medical Devices
Nowadays, a growing interest is centered on implantable and wearable medical devices with excellent translational potential in the clinic, like tissue scaffolds, biosensors, and biodetectors. However, it is crucial to establish conformal and stable contact between those devices and the target tissue (Schiavone and Lacour, 2019; Yuk et al., 2019). Wires and sutures are required for this fixation, which raises concerns of infection, secondary tissue injury, and scaffold damage. As a noninvasive adhesion method, bioadhesives have the potential to replace these invasive fixation methods. Based on a thin layer of a graphene nanocomposite, Deng et al. developed an electrical bioadhesive that can provide rapid, robust, and on-demand detachable integration of bioelectronic devices on diverse wet dynamic tissues (Deng et al., 2021). Later, they successfully used the e-bioadhesive to record an in situ epicardial electrocardiogram and electrically stimulated a sciatic nerve on a rat model. This technique offers a promising solution for addressing the long-standing challenges in tissue–device integration. Another good aspect of bioadhesives to be used in these situations is that different functions can be added into the bioadhesives to improve the outcome of the medical devices or reduce the potential complications. For example, the antibacterial property can be introduced to reduce the chance of medical devices’ infection (Hwang et al., 2018). In fact, there is still a vast area of bioadhesives in medical device fixation waiting to be explored. However, one should be careful because the bioadhesives may also adversely influence the medical devices. Macnab et al. showed that Tisseel® significantly attenuated NIR light of a near-infrared spectroscopy during in vitro transmittance and critically compromised photo transmission in vivo (Macnab et al., 2018). Another fixation method is also required when there is a need for tissue transplantation. Islet transplantation is used to treat type I diabetes by replacing the lost beta cell function. Brubaker et al. directly immobilized islets onto intra-abdominal tissue surfaces using a thin layer of a mussel-inspired bioadhesive (Brubaker et al., 2010). On the one hand, the fixation approach offers the potential advantages for convenient, rapid, and minimally invasive islet transplantation by direct apposition of the islet bolus onto tissue surfaces. On the other hand, the technique avoids the intravascular engraftment site, eliminating adverse effects of first-pass blood exposure in the liver while maintaining the capability of rapid islet revascularization and the benefits of direct insulin secretion into the portal circulation.
Perspective
Bioadhesives are believed to revolutionize the surgical process (Mehdizadeh and Yang, 2013; Taboada et al., 2020). They have already been widely used as adhesives and sealants in the clinic to reduce complications and improve outcomes. However, those commercialized products are still far from satisfactory. Fibrin-based, PEG-based, and cyanoacrylate-based bioadhesives are the most commonly used ones. Fibrin-based and PEG-based bioadhesives have good biocompatibility but weak adhesion strength. So, most of them are only used as adjunctions for traditional wound closure or sealing methods. Cyanoacrylate-based bioadhesives have strong adhesion strength, but their potential safety concerns limit their wide applications, especially internal applications. Thus, more powerful and commercially transformable bioadhesives for wound closure and sealing leakage are still needed.
Compared with traditional wound dressings, bioadhesives get easily attached to the parts where they are applied because of their intrinsic adhesion property (Li and Mooney., 2016). So, there is a growing interest in using bioadhesives as a functional wound dressing. This application is beneficial for tissues where the fixation of traditional wound dressing fails to work, like a beating heart and brittle brain (Lin et al., 2019). However, the absence of removability makes it hard for further wound care or dressing change, resulting in more potential troubles when mechanical debridement is involved. So controllably removable property is also explored for bioadhesives (Chen et al., 2020; Bu et al., 2019; Villa-Camacho et al., 2015; Konieczynska et al., 2016).
Using bioadhesives for the local delivery of functional items like drugs or cells is also a promising way to realize specialized and prolonged effectiveness. Compared with conventional hydrogel vehicles, bioadhesives can adhere to tissues, making them more stable in special tissues like the beating heart and esophagus (Lin et al., 2019). By mixing Tannic and PEG, Lee et al. developed a new medical glue called TAPE, which had been applied to the esophagus and demonstrated the ability to detect gastroesophageal reflux diseases because it maintained wet-adhesive properties (Kim et al., 2015). Bioadhesives are also used to fix medical devices or tissues, of which the importance is increasing with an increasing number of implantable medical devices and tissue transplantation. The fixation using bioadhesives will not damage either medical devices or the tissues. Although very promising, there is a difficulty in avoiding the interference between the functions of medical devices and bioadhesives. Besides, for tissue transplantation, the adhesion strength of bioadhesives available might not be sufficient for large pieces of tissues.
Although massive efforts have been spent on developing bioadhesives, there are only a handful of products available in the market (Taboada et al., 2020). First, the researcher might care too much about the adhesion mechanism, while cohesion is ignored. Cohesion dramatically influences how the bioadhesives would be used, which is particularly important for clinical translation. In the market, ease of use has a positive influence on people’s choices. Second, one bioadhesive never fits all the applications. The requirement of bioadhesives for wound closure differs from those for sealing leakage. So, it is suggested to choose the unmet clinical target first and then the relative characterization methods to fabricate bioadhesives for translation.
Author Contributions
WD did the literature search and paper writing. XB did the literature research and helped revise the paper. YB was responsible for the whole paper design and manuscript organization.
Conflict of Interest
The authors declare that the research was conducted in the absence of any commercial or financial relationships that could be construed as a potential conflict of interest.
Acknowledgments
We sincerely thank Abhay Pandit for offering inspiration and practical advice, as well as writing support for this publication. The study was funded by ‘Young Talent Support Plan’ of Xi’an Jiaotong University.
Publisher’s Note
All claims expressed in this article are solely those of the authors and do not necessarily represent those of their affiliated organizations, or those of the publisher, the editors and the reviewers. Any product that may be evaluated in this article, or claim that may be made by its manufacturer, is not guaranteed or endorsed by the publisher.
References
Annabi, N., Zhang, Y.-N., Assmann, A., Sani, E. S., Cheng, G., Lassaletta, A. D., et al. (2017). Engineering a Highly Elastic Human Protein-Based Sealant for Surgical Applications. Sci. Transl. Med. 9 (410), eaai7466. doi:10.1126/scitranslmed.aai7466
Artzi, N. (2013). Sticking with the Pattern for a Safer Glue. Sci. Translational Med. 5 (205), 205ec161. doi:10.1126/scitranslmed.3007663
Assmann, A., Vegh, A., Ghasemi-Rad, M., Bagherifard, S., Cheng, G., Sani, E. S., et al. (2017). A Highly Adhesive and Naturally Derived Sealant. Biomaterials 140, 115–127. doi:10.1016/j.biomaterials.2017.06.004
Azuma, K., Nishihara, M., Shimizu, H., Itoh, Y., Takashima, O., Osaki, T., et al. (2015). Biological Adhesive Based on Carboxymethyl Chitin Derivatives and Chitin Nanofibers. Biomaterials 42, 20–29. doi:10.1016/j.biomaterials.2014.11.043
Bae, W. G., Kim, D., Kwak, M. K., Ha, L., Kang, S. M., and Suh, K. Y. (2013). Enhanced Skin Adhesive Patch with Modulus-Tunable Composite Micropillars. Adv. Healthc. Mater. 2 (1), 109–113. doi:10.1002/adhm.201200098
Balakrishnan, B., Soman, D., Payanam, U., Laurent, A., Labarre, D., and Jayakrishnan, A. (2017). A Novel Injectable Tissue Adhesive Based on Oxidized Dextran and Chitosan. Acta Biomater. 53, 343–354. doi:10.1016/j.actbio.2017.01.065
Bao, Z., Gao, M., Sun, Y., Nian, R., and Xian, M. (2020). The Recent Progress of Tissue Adhesives in Design Strategies, Adhesive Mechanism and Applications. Mater. Sci. Eng. C 111, 110796. doi:10.1016/j.msec.2020.110796
Behrens, A. M., Lee, N. G., Casey, B. J., Srinivasan, P., Sikorski, M. J., Daristotle, J. L., et al. (2015). Biodegradable-Polymer-Blend-Based Surgical Sealant with Body-Temperature-Mediated Adhesion. Adv. Mater. 27 (48), 8056–8061. doi:10.1002/adma.201503691
Behrens, A. M., Sikorski, M. J., Li, T., Wu, Z. J., Griffith, B. P., and Kofinas, P. (2014). Blood-aggregating Hydrogel Particles for Use as a Hemostatic Agent. Acta Biomater. 10 (2), 701–708. doi:10.1016/j.actbio.2013.10.029
Blacklow, S. O., Lisker, S., Ng, M. Y., Sarkar, U., and Lyles, C. (2019). Bioinspired Mechanically Active Adhesive Dressings to Accelerate Wound Closure. Sci. Adv. 5 (7), eaaw3963. doi:10.1126/sciadv.aaw3963
Brubaker, C. E., Kissler, H., Wang, L.-J., Kaufman, D. B., and Messersmith, P. B. (2010). Biological Performance of Mussel-Inspired Adhesive in Extrahepatic Islet Transplantation. Biomaterials 31 (3), 420–427. doi:10.1016/j.biomaterials.2009.09.062
Bu, Y.-z., Sun, G.-f., Zhang, L.-c., Liu, J.-h., Yang, F., Tang, P.-f., et al. (2017). POSS-modified PEG Adhesives for Wound Closure. Chin J. Polym. Sci. 35 (10), 1231–1242. doi:10.1007/s10118-017-1958-x
Bu, Y., Wang, X., Li, L., Hu, X., Tan, D., Li, Z., et al. (2020). Lithium Loaded Octa‐Poly(Ethylene Glycol) Based Adhesive Facilitates Axon Regeneration and Reconnection of Transected Peripheral Nerves. Adv. Healthc. Mater. 9 (13), 2000268. doi:10.1002/adhm.202000268
Bu, Y., Zhang, L., Liu, J., Zhang, L., Li, T., Shen, H., et al. (2016). Synthesis and Properties of Hemostatic and Bacteria-Responsive In Situ Hydrogels for Emergency Treatment in Critical Situations. ACS Appl. Mater. Inter. 8 (20), 12674–12683. doi:10.1021/acsami.6b03235
Bu, Y., Zhang, L., Sun, G., Sun, F., Liu, J., Yang, F., et al. (2019). Tetra‐PEG Based Hydrogel Sealants for In Vivo Visceral Hemostasis. Adv. Mater. 31 (28), 1901580. doi:10.1002/adma.201901580
Cao, J., Xiao, L., and Shi, X. (2019). Injectable Drug-Loaded Polysaccharide Hybrid Hydrogels for Hemostasis. RSC Adv. 9 (63), 36858–36866. doi:10.1039/c9ra07116d
Cedano Serrano, F. J., Pinzón, L. M., Narváez, D. M., Castro Paéz, C. I., Moreno-Serrano, C. L., Tabima, D. M., et al. (2017). Evaluation of a Water-Resistant and Biocompatible Adhesive with Potential Use in Bone Fractures. J. Adhes. Sci. Technology 31 (13), 1480–1495. doi:10.1080/01694243.2016.1263055
Chan Choi, Y., Choi, J. S., Jung, Y. J., and Cho, Y. W. (2014). Human Gelatin Tissue-Adhesive Hydrogels Prepared by Enzyme-Mediated Biosynthesis of DOPA and Fe3+ion Crosslinking. J. Mater. Chem. B 2 (2), 201–209. doi:10.1039/c3tb20696c
Chang, E. I., Galvez, M. G., Glotzbach, J. P., Hamou, C. D., El-ftesi, S., Rappleye, C. T., et al. (2011). Vascular Anastomosis Using Controlled Phase Transitions in Poloxamer Gels. Nat. Med. 17 (9), 1147–1152. doi:10.1038/nm.2424
Chen, W., Wang, R., Xu, T., Ma, X., Yao, Z., Chi, B., et al. (2017). A Mussel-Inspired Poly(γ-Glutamic Acid) Tissue Adhesive with High Wet Strength for Wound Closure. J. Mater. Chem. B 5 (28), 5668–5678. doi:10.1039/c7tb00813a
Chen, X., Yuk, H., Wu, J., Nabzdyk, C. S., and Zhao, X. (2020). Instant Tough Bioadhesive with Triggerable Benign Detachment. Proc. Natl. Acad. Sci. USA 117 (27), 15497–15503. doi:10.1073/pnas.2006389117
Chen, Y., Cheng, W., Teng, L., Jin, M., Lu, B., Ren, L., et al. (2018). Graphene Oxide Hybrid Supramolecular Hydrogels with Self-Healable, Bioadhesive and Stimuli-Responsive Properties and Drug Delivery Application. Macromol. Mater. Eng. 303 (8), 1700660. doi:10.1002/mame.201700660
Cui, C., Fan, C., Wu, Y., Xiao, M., Wu, T., Zhang, D., et al. (2019). Water‐Triggered Hyperbranched Polymer Universal Adhesives: From Strong Underwater Adhesion to Rapid Sealing Hemostasis. Adv. Mater. 31 (49), 1905761. doi:10.1002/adma.201905761
Cui, C., Wu, T., Gao, F., Fan, C., Xu, Z., Wang, H., et al. (2018). An Autolytic High Strength Instant Adhesive Hydrogel for Emergency Self-rescue. Adv. Funct. Mater. 28 (42), 1804925. doi:10.1002/adfm.201804925
Deng, J., Tang, Y., Zhang, Q., Wang, C., Liao, M., Ji, P., et al. (2019). A Bioinspired Medical Adhesive Derived from Skin Secretion of andrias Davidianus for Wound Healing. Adv. Funct. Mater. 29 (31), 1809110. doi:10.1002/adfm.201809110
Deng, J., Yuk, H., Wu, J., Varela, C. E., Chen, X., Roche, E. T., et al. (2021). Electrical Bioadhesive Interface for Bioelectronics. Nat. Mater. 20 (2), 229–236. doi:10.1038/s41563-020-00814-2
Dowling, M. B., Kumar, R., Keibler, M. A., Hess, J. R., Bochicchio, G. V., and Raghavan, S. R. (2011). A Self-Assembling Hydrophobically Modified Chitosan Capable of Reversible Hemostatic Action. Biomaterials 32 (13), 3351–3357. doi:10.1016/j.biomaterials.2010.12.033
Du, X., Wu, L., Yan, H., Qu, L., Wang, L., Wang, X., et al. (2019). Multifunctional Hydrogel Patch with Toughness, Tissue Adhesiveness, and Antibacterial Activity for Sutureless Wound Closure. ACS Biomater. Sci. Eng. 5 (5), 2610–2620. doi:10.1021/acsbiomaterials.9b00130
Elvin, C. M., Vuocolo, T., Brownlee, A. G., Sando, L., Huson, M. G., Liyou, N. E., et al. (2010). A Highly Elastic Tissue Sealant Based on Photopolymerised Gelatin. Biomaterials 31 (32), 8323–8331. doi:10.1016/j.biomaterials.2010.07.032
Farrar, D. F. (2012). Bone Adhesives for Trauma Surgery: A Review of Challenges and Developments. Int. J. Adhes. Adhesives 33, 89–97. doi:10.1016/j.ijadhadh.2011.11.009
Feng, Q., Wei, K., Lin, S., Xu, Z., Sun, Y., Shi, P., et al. (2016). Mechanically Resilient, Injectable, and Bioadhesive Supramolecular Gelatin Hydrogels Crosslinked by Weak Host-Guest Interactions Assist Cell Infiltration and In Situ Tissue Regeneration. Biomaterials 101, 217–228. doi:10.1016/j.biomaterials.2016.05.043
Fonouni, H., Kashfi, A., Stahlheber, O., Konstantinidis, L., Kraus, T. W., Mehrabi, A., et al. (2017). Analysis of the Biliostatic Potential of Two Sealants in a Standardized Porcine Model of Liver Resection. Am. J. Surg. 214 (5), 945–955. doi:10.1016/j.amjsurg.2017.06.038
Gan, D., Xing, W., Jiang, L., Fang, J., Zhao, C., Ren, F., et al. (2019). Plant-inspired Adhesive and Tough Hydrogel Based on Ag-Lignin Nanoparticles-Triggered Dynamic Redox Catechol Chemistry. Nat. Commun. 10 (1), 1487. doi:10.1038/s41467-019-09351-2
Ge, L., and Chen, S. (2020). Recent Advances in Tissue Adhesives for Clinical Medicine. Polymers 12 (4), 939. doi:10.3390/polym12040939
Giano, M. C., Ibrahim, Z., Medina, S. H., Sarhane, K. A., Christensen, J. M., Yamada, Y., et al. (2014). Injectable Bioadhesive Hydrogels with Innate Antibacterial Properties. Nat. Commun. 5, 4095. doi:10.1038/ncomms5095
Gong, C., Lu, C., Li, B., Shan, M., and Wu, G. (2017). Injectable Dopamine-Modified Poly(α,β-Aspartic Acid) Nanocomposite Hydrogel as Bioadhesive Drug Delivery System. J. Biomed. Mater. Res. 105 (4), 1000–1008. doi:10.1002/jbm.a.35931
Han, H.-K., Shin, H.-J., and Ha, D. H. (2012). Improved Oral Bioavailability of Alendronate via the Mucoadhesive Liposomal Delivery System. Eur. J. Pharm. Sci. 46 (5), 500–507. doi:10.1016/j.ejps.2012.04.002
Han, L., Lu, X., Liu, K., Wang, K., Fang, L., Weng, L.-T., et al. (2017). Mussel-inspired Adhesive and Tough Hydrogel Based on Nanoclay Confined Dopamine Polymerization. ACS Nano 11 (3), 2561–2574. doi:10.1021/acsnano.6b05318
Han, L., Wang, M., Li, P., Gan, D., Yan, L., Xu, J., et al. (2018). Mussel-inspired Tissue-Adhesive Hydrogel Based on the Polydopamine-Chondroitin Sulfate Complex for Growth-factor-free Cartilage Regeneration. ACS Appl. Mater. Inter. 10 (33), 28015–28026. doi:10.1021/acsami.8b05314
Han, L., Yan, L., Wang, K., Fang, L., Zhang, H., Tang, Y., et al. (2017). Tough, Self-Healable and Tissue-Adhesive Hydrogel with Tunable Multifunctionality. NPG Asia Mater. 9 (4), e372. doi:10.1038/am.2017.33
Harsha, K. S. S., and Vasudha, P. (2018). Cyanoacrylates: An Overview in its Application as a Tissue Adhesive. Der Pharma Chemica 10 (8), 42–46.
Hasani-Sadrabadi, M. M., Sarrion, P., Pouraghaei, S., Chau, Y., Ansari, S., Li, S., et al. (2020). An Engineered Cell-Laden Adhesive Hydrogel Promotes Craniofacial Bone Tissue Regeneration in Rats. Sci. Translational Med. 12 (534), eaay6853. doi:10.1126/scitranslmed.aay6853
Hong, S., Pirovich, D., Kilcoyne, A., Huang, C.-H., Lee, H., and Weissleder, R. (2016). Supramolecular Metallo-Bioadhesive for Minimally Invasive Use. Adv. Mater. 28 (39), 8675–8680. doi:10.1002/adma.201602606
Hong, Y., Zhou, F., Hua, Y., Zhang, X., Ni, C., Pan, D., et al. (2019). A Strongly Adhesive Hemostatic Hydrogel for the Repair of Arterial and Heart Bleeds. Nat. Commun. 10 (1), 2060. doi:10.1038/s41467-019-10004-7
Hu, J. K., Suh, H. W., Qureshi, M., Lewis, J. M., Yaqoob, S., Moscato, Z. M., et al. (2021). Nonsurgical Treatment of Skin Cancer with Local Delivery of Bioadhesive Nanoparticles. Proc. Natl. Acad. Sci. 118 (7), e2020575118. doi:10.1073/pnas.2020575118
Hwang, I., Kim, H. N., Seong, M., Lee, S.-H., Kang, M., Yi, H., et al. (2018). Multifunctional Smart Skin Adhesive Patches for Advanced Health Care. Adv. Healthc. Mater. 7 (15), 1800275. doi:10.1002/adhm.201800275
Jeon, E. Y., Hwang, B. H., Yang, Y. J., Kim, B. J., Choi, B.-H., Jung, G. Y., et al. (2015). Rapidly Light-Activated Surgical Protein Glue Inspired by Mussel Adhesion and Insect Structural Crosslinking. Biomaterials 67, 11–19. doi:10.1016/j.biomaterials.2015.07.014
Khademhosseini, A., and Langer, R. (2016). A Decade of Progress in Tissue Engineering. Nat. Protoc. 11 (10), 1775–1781. doi:10.1038/nprot.2016.123
Kim, H. J., Hwang, B. H., Lim, S., Choi, B.-H., Kang, S. H., and Cha, H. J. (2015a). Mussel Adhesion-Employed Water-Immiscible Fluid Bioadhesive for Urinary Fistula Sealing. Biomaterials 72, 104–111. doi:10.1016/j.biomaterials.2015.08.055
Kim, K., Ryu, J. H., Koh, M. Y., Yun, S. P., Kim, S., Park, J. P., et al. (2021). Coagulopathy-independent, Bioinspired Hemostatic Materials: A Full Research story from Preclinical Models to a Human Clinical Trial. Sci. Adv. 7 (13), eabc9992. doi:10.1126/sciadv.abc9992
Kim, K., Shin, M., Koh, M.-Y., Ryu, J. H., Lee, M. S., Hong, S., et al. (2015b). TAPE: A Medical Adhesive Inspired by a Ubiquitous Compound in Plants. Adv. Funct. Mater. 25 (16), 2402–2410. doi:10.1002/adfm.201500034
Kim, S.-H., Kim, K., Kim, B. S., An, Y.-H., Lee, U.-J., Lee, S.-H., et al. (2020). Fabrication of Polyphenol-Incorporated Anti-inflammatory Hydrogel via High-Affinity Enzymatic Crosslinking for Wet Tissue Adhesion. Biomaterials 242, 119905. doi:10.1016/j.biomaterials.2020.119905
Konieczynska, M. D., Villa-Camacho, J. C., Ghobril, C., Perez-Viloria, M., Tevis, K. M., Blessing, W. A., et al. (2016). On-demand Dissolution of a Dendritic Hydrogel-Based Dressing for Second-Degree Burn Wounds through Thiol-Thioester Exchange Reaction. Angew. Chem. Int. Ed. 55 (34), 9984–9987. doi:10.1002/anie.201604827
Krishnadoss, V., Melillo, A., Kanjilal, B., Hannah, T., Ellis, E., Kapetanakis, A., et al. (2019). Bioionic Liquid Conjugation as Universal Approach to Engineer Hemostatic Bioadhesives. ACS Appl. Mater. Inter. 11 (42), 38373–38384. doi:10.1021/acsami.9b08757
Lang, N., Pereira, M. J., Lee, Y., Friehs, I., Vasilyev, N. V., Feins, E. N., et al. (2014). A Blood-Resistant Surgical Glue for Minimally Invasive Repair of Vessels and Heart Defects. Sci. Translational Med. 6 (218), 218ra6. doi:10.1126/scitranslmed.3006557
Li, J., and Mooney, D. J. (2016). Designing Hydrogels for Controlled Drug Delivery. Nat. Rev. Mater. 1 (12), 1–17. doi:10.1038/natrevmats.2016.71
Li, S., Zhou, J., Huang, Y., Roy, J., Zhou, N., Yum, K., et al. (2020). Injectable Click Chemistry-Based Bioadhesives for Accelerated Wound Closure. Acta Biomater. 110, 95–104. doi:10.1016/j.actbio.2020.04.004
Liang, Y., Zhao, X., Hu, T., Chen, B., Yin, Z., Ma, P. X., et al. (2019). Adhesive Hemostatic Conducting Injectable Composite Hydrogels with Sustained Drug Release and Photothermal Antibacterial Activity to Promote Full‐Thickness Skin Regeneration during Wound Healing. Small 15 (12), 1900046. doi:10.1002/smll.201900046
Lih, E., Lee, J. S., Park, K. M., and Park, K. D. (2012). Rapidly Curable Chitosan-PEG Hydrogels as Tissue Adhesives for Hemostasis and Wound Healing. Acta Biomater. 8 (9), 3261–3269. doi:10.1016/j.actbio.2012.05.001
Lin, X., Liu, Y., Bai, A., Cai, H., Bai, Y., Jiang, W., et al. (2019). A Viscoelastic Adhesive Epicardial Patch for Treating Myocardial Infarction. Nat. Biomed. Eng. 3 (8), 632–643. doi:10.1038/s41551-019-0380-9
Liu, B., Wang, Y., Miao, Y., Zhang, X., Fan, Z., Singh, G., et al. (2018). Hydrogen Bonds Autonomously Powered Gelatin Methacrylate Hydrogels with Super-elasticity, Self-Heal and Underwater Self-Adhesion for Sutureless Skin and Stomach Surgery and E-Skin. Biomaterials 171, 83–96. doi:10.1016/j.biomaterials.2018.04.023
Liu, C., Liu, X., Liu, C., Wang, N., Chen, H., Yao, W., et al. (2019). A Highly Efficient, In Situ Wet-Adhesive Dextran Derivative Sponge for Rapid Hemostasis. Biomaterials 205, 23–37. doi:10.1016/j.biomaterials.2019.03.016
Lu, M., Liu, Y., Huang, Y.-C., Huang, C.-J., and Tsai, W.-B. (2018). Fabrication of Photo-Crosslinkable Glycol Chitosan Hydrogel as a Tissue Adhesive. Carbohydr. Polym. 181, 668–674. doi:10.1016/j.carbpol.2017.11.097
Lu, X., Shi, S., Li, H., Gerhard, E., Lu, Z., Tan, X., et al. (2020). Magnesium Oxide-Crosslinked Low-Swelling Citrate-Based Mussel-Inspired Tissue Adhesives. Biomaterials 232, 119719. doi:10.1016/j.biomaterials.2019.119719
Macnab, A., Pagano, R., Kwon, B., Dumont, G., and Shadgan, B. (2018). “In Vivo Near Infrared (NIRS) Sensor Attachment Using Fibrin Bioadhesive”, in Proceedings Volume 10501, Optical Diagnostics and Sensing XVIII: Toward Point-of-Care Diagnostics (San Francisco, California, United States: SPIE BiOS), 1–8.
Margules, A. C., and Rovner, E. S. (2019). The Use of Tissue Flaps in the Management of Urinary Tract Fistulas. Curr. Urol. Rep. 20 (6), 32. doi:10.1007/s11934-019-0892-6
Mehdizadeh, M., Weng, H., Gyawali, D., Tang, L., and Yang, J. (2012). Injectable Citrate-Based Mussel-Inspired Tissue Bioadhesives with High Wet Strength for Sutureless Wound Closure. Biomaterials 33 (32), 7972–7983. doi:10.1016/j.biomaterials.2012.07.055
Mehdizadeh, M., and Yang, J. (2013). Design Strategies and Applications of Tissue Bioadhesives. Macromol. Biosci. 13 (3), 271–288. doi:10.1002/mabi.201200332
Mueller, M. R., and Marzluf, B. A. (2014). The Anticipation and Management of Air Leaks and Residual Spaces post Lung Resection. J. Thorac. Dis. 6 (3), 271–284. doi:10.3978/j.issn.2072-1439.2013.11.29
Nie, W., Yuan, X., Zhao, J., Zhou, Y., and Bao, H. (2013). Rapidly In Situ Forming Chitosan/ε-Polylysine Hydrogels for Adhesive Sealants and Hemostatic Materials. Carbohydr. Polym. 96 (1), 342–348. doi:10.1016/j.carbpol.2013.04.008
Patel, R. G., Purwada, A., Cerchietti, L., Inghirami, G., Melnick, A., Gaharwar, A. K., et al. (2014). Microscale Bioadhesive Hydrogel Arrays for Cell Engineering Applications. Cel. Mol. Bioeng. 7 (3), 394–408. doi:10.1007/s12195-014-0353-8
Pathak, K., and Malviya, R. (2020). Introduction, Theories and Mechanisms of Bioadhesion, Bioadhesives in Drug Delivery, 1–27. doi:10.1002/9781119640240.ch1
Pausch, T. M., Mitzscherling, C., Abbasi, S., Cui, J., Liu, X., Aubert, O., et al. (2020). SmartPAN: A Novel Polysaccharide-Microsphere-Based Surgical Indicator of Pancreatic Leakage. J. Biomater. Appl. 35 (1), 123–134. doi:10.1177/0885328220913057
Qu, J., Zhao, X., Liang, Y., Zhang, T., Ma, P. X., and Guo, B. (2018). Antibacterial Adhesive Injectable Hydrogels with Rapid Self-Healing, Extensibility and Compressibility as Wound Dressing for Joints Skin Wound Healing. Biomaterials 183, 185–199. doi:10.1016/j.biomaterials.2018.08.044
Reddy, P. C., Chaitanya, KS., and Madhusudan Rao, Y. (2011). A Review on Bioadhesive Buccal Drug Delivery Systems: Current Status of Formulation and Evaluation Methods. DARU J. Pharm. Sci. 19 (6), 385.
Ryu, J. H., Lee, Y., Kong, W. H., Kim, T. G., Park, T. G., and Lee, H. (2011). Catechol-functionalized Chitosan/Pluronic Hydrogels for Tissue Adhesives and Hemostatic Materials. Biomacromolecules 12 (7), 2653–2659. doi:10.1021/bm200464x
Schiavone, G., and Lacour, SP. (2019). Conformable Bioelectronic Interfaces: Mapping the Road Ahead. Sci. Translational Med. 11, eaaw5858. doi:10.1126/scitranslmed.aaw5858
Shin, J., Lee, J. S., Lee, C., Park, H.-J., Yang, K., Jin, Y., et al. (2015). Tissue Adhesive Catechol-Modified Hyaluronic Acid Hydrogel for Effective, Minimally Invasive Cell Therapy. Adv. Funct. Mater. 25 (25), 3814–3824. doi:10.1002/adfm.201500006
Shin, M., Kim, K., Shim, W., Yang, J. W., and Lee, H. (2016). Tannic Acid as a Degradable Mucoadhesive Compound. ACS Biomater. Sci. Eng. 2 (4), 687–696. doi:10.1021/acsbiomaterials.6b00051
Shin, M., Park, S.-G., Oh, B.-C., Kim, K., Jo, S., Lee, M. S., et al. (2016). Complete Prevention of Blood Loss with Self-Sealing Haemostatic needles. Nat. Mater 16, 147–152. doi:10.1038/nmat4758
Shin, M., Ryu, J. H., Park, J. P., Kim, K., Yang, J. W., and Lee, H. (2015). DNA/Tannic Acid Hybrid Gel Exhibiting Biodegradability, Extensibility, Tissue Adhesiveness, and Hemostatic Ability. Adv. Funct. Mater. 25 (8), 1270–1278. doi:10.1002/adfm.201403992
Shirzaei Sani, E., Kheirkhah, A., Rana, D., Sun, Z., Foulsham, W., Sheikhi, A., et al. (2019). Sutureless Repair of Corneal Injuries Using Naturally Derived Bioadhesive Hydrogels. Sci. Adv. 5 (3), eaav1281. doi:10.1126/sciadv.aav1281
Slieker, J. C., Daams, F., Mulder, I. M., Jeekel, J., and Lange, J. F. (2013). Systematic Review of the Technique of Colorectal Anastomosis. JAMA Surg. 148 (2), 190–201. doi:10.1001/2013.jamasurg.33
Spotnitz, W. D., and Burks, S. (2012). Hemostats, Sealants, and Adhesives III: A New Update as Well as Cost and Regulatory Considerations for Components of the Surgical Toolbox. Transfusion 52 (10), 2243–2255. doi:10.1111/j.1537-2995.2012.03707.x
Sun, F., Bu, Y., Chen, Y., Yang, F., Yu, J., and Wu, D. (2020). An Injectable and Instant Self-Healing Medical Adhesive for Wound Sealing. ACS Appl. Mater. Inter. 12 (8), 9132–9140. doi:10.1021/acsami.0c01022
Taboada, G. M., Yang, K., Pereira, M. J. N., Liu, S. S., Hu, Y., Karp, J. M., et al. (2020). Overcoming the Translational Barriers of Tissue Adhesives. Nat. Rev. Mater. 5 (4), 310–329. doi:10.1038/s41578-019-0171-7
Tan, H., Jin, D., Qu, X., Liu, H., Chen, X., Yin, M., et al. (2019). A PEG-Lysozyme Hydrogel Harvests Multiple Functions as a Fit-To-Shape Tissue Sealant for Internal-Use of Body. Biomaterials 192, 392–404. doi:10.1016/j.biomaterials.2018.10.047
Villa-Camacho, J. C., Ghobril, C., Anez-Bustillos, L., Grinstaff, M. W., Rodríguez, E. K., and Nazarian, A. (2015). The Efficacy of a Lysine-Based Dendritic Hydrogel Does Not Differ from Those of Commercially Available Tissue Sealants and Adhesives: An Ex Vivo Study. BMC Musculoskelet. Disord. 16 (116), 1–6. doi:10.1186/s12891-015-0573-7
Walker, B. W., Lara, R. P., Yu, C. H., Sani, E. S., Kimball, W., Joyce, S., et al. (2019). Engineering a Naturally-Derived Adhesive and Conductive Cardiopatch. Biomaterials 207, 89–101. doi:10.1016/j.biomaterials.2019.03.015
Wijetunge, S. S., Wen, J., Yeh, C.-K., and Sun, Y. (2018). Lectin-conjugated Liposomes as Biocompatible, Bioadhesive Drug Carriers for the Management of Oral Ulcerative Lesions. ACS Appl. Bio Mater. 1 (5), 1487–1495. doi:10.1021/acsabm.8b00425
Xie, D., Guo, J., Mehdizadeh, M. R., Tran, R. T., Chen, R., Sun, D., et al. (2015). Development of Injectable Citrate-Based Bioadhesive Bone Implants. J. Mater. Chem. B 3 (3), 387–398. doi:10.1039/c4tb01498g
Yan, S., Wang, W., Li, X., Ren, J., Yun, W., Zhang, K., et al. (2018). Preparation of Mussel-Inspired Injectable Hydrogels Based on Dual-Functionalized Alginate with Improved Adhesive, Self-Healing, and Mechanical Properties. J. Mater. Chem. B 6 (40), 6377–6390. doi:10.1039/c8tb01928b
Yanagihara, T., Maki, N., Wijesinghe, A. I., Sato, S., Saeki, Y., Kitazawa, S., et al. (2021). Efficacy of Alaska pollock Gelatin Sealant for Pulmonary Air Leakage in Porcine Models. Ann. Thorac. Surg. S0003-4975 (21), 00978-4. doi:10.1016/j.athoracsur.2021.05.023
Yang, S. Y., O'Cearbhaill, E. D., Sisk, G. C., Park, K. M., Cho, W. K., Villiger, M., et al. (2013). A Bio-Inspired Swellable Microneedle Adhesive for Mechanical Interlocking with Tissue. Nat. Commun. 4, 1702. doi:10.1038/ncomms2715
Yang, Y., Zhao, X., Yu, J., Chen, X., Wang, R., Zhang, M., et al. (2021). Bioactive Skin-Mimicking Hydrogel Band-Aids for Diabetic Wound Healing and Infectious Skin Incision Treatment. Bioactive Mater. 6 (11), 3962–3975. doi:10.1016/j.bioactmat.2021.04.007
Yuk, H., Lu, B., and Zhao, X. (2019). Hydrogel Bioelectronics. Chem. Soc. Rev. 48 (6), 1642–1667. doi:10.1039/c8cs00595h
Yuk, H., Varela, C. E., Nabzdyk, C. S., Mao, X., Padera, R. F., Roche, E. T., et al. (2019). Dry Double-Sided Tape for Adhesion of Wet Tissues and Devices. Nature 575 (7781), 169–174. doi:10.1038/s41586-019-1710-5
Zhang, C., Tang, J., Liu, D., Li, X., Cheng, L., and Tang, X. (2016). Design and Evaluation of an Innovative Floating and Bioadhesive Multiparticulate Drug Delivery System Based on Hollow Structure. Int. J. Pharmaceutics 503 (1-2), 41–55. doi:10.1016/j.ijpharm.2016.02.045
Zhang, L., Zuo, X., Li, S., Sun, M., Xie, H., Zhang, K., et al. (2019). Synergistic Therapy of Magnetism-Responsive Hydrogel for Soft Tissue Injuries. Bioactive Mater. 4, 160–166. doi:10.1016/j.bioactmat.2019.03.002
Zhang, Y., Yu, Y., Li, G., Meng, H., Zhang, X., Dong, L., et al. (2020). A Bioadhesive Nanoplatform Enhances the Permeation of Drugs Used to Treat Diabetic Macular Edema. ACS Appl. Bio Mater. 3 (4), 2314–2324. doi:10.1021/acsabm.0c00080
Zhao, X., Liang, Y., Huang, Y., He, J., Han, Y., and Guo, B. (2020). Physical Double‐Network Hydrogel Adhesives with Rapid Shape Adaptability, Fast Self‐Healing, Antioxidant and NIR/pH Stimulus‐Responsiveness for Multidrug‐Resistant Bacterial Infection and Removable Wound Dressing. Adv. Funct. Mater. 30 (17), 1910748. doi:10.1002/adfm.201910748
Zhao, X., Wu, H., Guo, B., Dong, R., Qiu, Y., and Ma, P. X. (2017). Antibacterial Anti-oxidant Electroactive Injectable Hydrogel as Self-Healing Wound Dressing with Hemostasis and Adhesiveness for Cutaneous Wound Healing. Biomaterials 122, 34–47. doi:10.1016/j.biomaterials.2017.01.011
Zhu, W., Chuah, Y. J., and Wang, D.-A. (2018). Bioadhesives for Internal Medical Applications: A Review. Acta Biomater. 74, 1–16. doi:10.1016/j.actbio.2018.04.034
Keywords: bioadhesive, sealant, wound closure, functional wound dressing, medical device fixation
Citation: Duan W, Bian X and Bu Y (2021) Applications of Bioadhesives: A Mini Review. Front. Bioeng. Biotechnol. 9:716035. doi: 10.3389/fbioe.2021.716035
Received: 28 May 2021; Accepted: 22 July 2021;
Published: 03 September 2021.
Edited by:
Francesco Baino, Politecnico di Torino, ItalyReviewed by:
Kyung Min Park, Incheon National University, South KoreaAshley Carson Brown, North Carolina State University, United States
Copyright © 2021 Duan, Bian and Bu. This is an open-access article distributed under the terms of the Creative Commons Attribution License (CC BY). The use, distribution or reproduction in other forums is permitted, provided the original author(s) and the copyright owner(s) are credited and that the original publication in this journal is cited, in accordance with accepted academic practice. No use, distribution or reproduction is permitted which does not comply with these terms.
*Correspondence: Yazhong Bu, yazhongbu@xjtu.edu.cn
†These authors have contributed equally to this work