Development of the Pectoral Lobed Fin in the Australian Lungfish Neoceratodus forsteri
- 1Department of Earth and Planetary Science, Graduate School of Science, The University of Tokyo, Tokyo, Japan
- 2Departamento de Zoologia, Instituto de Biologia/IBRAG, Universidade do Estado do Rio de Janeiro, Rio de Janeiro, Brazil
- 3Kitakyushu Museum of Natural History and Human History, Kitakyushu, Japan
- 4Department of Anatomy, Iwate Medical University School of Medicine, Morioka, Japan
- 5Japan Synchrotron Radiation Research Institute, Sayo, Japan
The evolutionary transition from paired fins to limbs involved the establishment of a set of limb muscles as an evolutionary novelty. In parallel, there was a change in the topography of the spinal nerves innervating appendicular muscles, so that distinct plexuses were formed at the bases of limbs. However, the key developmental changes that brought about this evolutionary novelty have remained elusive due to a lack of data on the development of lobed fins in sarcopterygian fishes. Here, we observed the development of the pectoral fin in the Australian lungfish Neoceratodus forsteri (Sarcopterygii) through synchrotron radiation X-ray microtomography. Neoceratodus forsteri is a key taxon for understanding the fin-to-limb transition due to its close phylogenetic relationships to tetrapods and well-developed lobed fins. At the onset of the fin bud in N. forsteri, there is no mesenchyme at the junction between the axial body wall and the fin bud, which corresponds to the embryonic position of the brachial plexus formed in the mesenchyme in tetrapods. Later, concurrent with the cartilage formation in the fin skeleton, the fin adductor and abductor muscles become differentiated within the surface ectoderm of the fin bud. Subsequently, the girdle muscle, which is homologous to the tetrapod serratus muscle, newly develops at the junction between the axial body wall and the fin. Our study suggests that the acquisition of embryonic mesenchyme at the junction between the axial body wall and the appendicular bud opened the door to the formation of the brachial plexus and the specialization of individual muscles in the lineage that gave rise to tetrapods.
Introduction
One of the pivotal challenges of the animals’ water-to-land transition is the difficulty in weight support and locomotion on land under gravity constraints. The tetrapod limbs had evolved from paired fins during the Devonian (Ahlberg, 2019), and were decisive for the success of this transition. Although fossil transitional forms from the fin to the limb potentially bridge the gap, tetrapod limbs differ from paired fins in mode of evolutionary change, or variational modality, thereby representing an evolutionary novelty (Wagner, 2014). The origin of this novelty involved the functional integration of the musculoskeletal and nervous systems necessary for terrestrial and aerial movement, and represents one of the most drastic morphological changes in vertebrate evolution.
Attempts to understand the evolution of the limb and the developmental basis of the morphological transition have involved comparative anatomy, paleontology, and evolutionary developmental biology. Since soft-tissues are poorly preserved in the fossil record, much emphasis is currently placed on the skeletal systems. In particular, the evolutionary origin of the digit and wrist (i.e., the autopod) has been gradually unraveled through paleontological studies of Devonian fossils (Shubin et al., 2006; Cloutier et al., 2020) and through evolutionary developmental studies focused mainly on the 5’Hox genes expressed in fin and limb buds (Shubin and Alberch, 1986; Davis et al., 2007; Johanson et al., 2007; Nakamura et al., 2016; Tanaka, 2016; Woltering et al., 2020). These studies have dispelled any uncertainty about the homologies of proximal (i.e., the stylopod and zeugopod) skeletal elements in fins and limbs, and the morphological transitions from one to the other have been traced in relation to the evolution of tetrapod limb movement (Shubin et al., 2004, 2006; Pierce et al., 2012; Miyake et al., 2016; Molnar et al., 2017; Ahlberg, 2019; Wynd et al., 2019).
Despite this progress, however, the process of the soft-tissue evolution remains elusive. So far, morphologies of the muscles (Braus, 1901; Shann, 1920, 1924; Diogo et al., 2016; Miyake et al., 2016; Molnar et al., 2017, 2018), spinal nerves (Fürbringer, 1888; Braus, 1901; Hirasawa and Kuratani, 2018), and vascular system (Ura, 1956; Saito, 1988a,b) have been compared between fins and limbs, but their homologies are still, at least partly, uncertain. In fossil taxa, data about muscle attachments can provide clues for muscle morphologies (Sanchez et al., 2013; Molnar et al., 2017), but it is quite difficult to capture the complete picture of the musculoskeletal system of non-tetrapodomorph and tetrapodomorph fishes, as well as early tetrapods. The extant tetrapods possess a common set of limb muscles and corresponding spinal nerves, and the topographies of both systems have remained largely consistent during the evolution of tetrapod crown groups. Recent studies on the evolution of limb muscles (Molnar et al., 2017, 2018) suggest that the full set of limb muscles was acquired in a stepwise manner in the early evolution of limbed tetrapods. Also, the plexus of spinal nerves formed at the base of the limb is likely an evolutionary novelty in the tetrapod lineage (Hirasawa and Kuratani, 2018). Formation of the limb-innervating plexus potentially facilitated the complex control of movement by the columnar organization of neurons in the spinal cord (i.e., the lateral motor column) (Murakami and Tanaka, 2011; Jung et al., 2018), by enabling axons from neurons at different cranio-caudal levels to innervate a single muscle together.
From the developmental point of view, modes of migration of somite-derived muscle progenitor cells have been compared between tetrapods and fishes, in particular elasmobranchs, whose fin muscles are arranged in parallel to the somites (Okamoto et al., 2017; Turner et al., 2019). Although data on the mode of limb muscle development in lissamphibians remains insufficient, at least for amniotes there is ample evidence that the limb muscle progenitor cells dissolve their somitic segmentation patterns upon entrance to the limb bud (Dietrich et al., 1998; Gross et al., 2000; Hirasawa and Kuratani, 2018). Almost simultaneously with the migration of muscle progenitor cells into the limb bud, the developing spinal nerves form the brachial plexus within the mesenchyme at the junction between the axial body wall and the limb bud (i.e., the plexus mesenchyme), which is required for normal development of nerves innervating limb muscles (Wright and Snider, 1996; Haase et al., 2002; Kramer et al., 2006). This dissolution of the segmentation pattern of the musculature and spinal nerves at the junction between the axial body wall and limb bud may represent a developmental process required for the establishment of limb muscles in extant tetrapods. On the other hand, based on the descriptions of pectoral fin development in the sturgeon (Mollier, 1897) and zebrafish (Grandel and Schulte-Merker, 1998), no distinct mesenchyme develops at the junction between the axial body wall and fin bud in these actinopterygian fishes.
Because the tetrapod limb evolved from the lobed fin of ancestral sarcopterygian fishes, the development of lobed fins in extant non-tetrapod sarcopterygians including coelacanths and lungfishes potentially provides clues to understanding key evolutionary changes in muscle and nerve development. Among the extant non-tetrapod sarcopterygians, in which lungfishes are closer to tetrapods than coelacanths (Amemiya et al., 2013; Betancur-R et al., 2017), the Australian lungfish Neoceratodus forsteri provides the best model for developmental studies of lobe-finned fishes; other species present problems either because of the inaccessibility of large embryonic samples (e.g., coelacanths) or secondary modifications in fin morphology (e.g., African and South American lungfishes, having undeveloped filamentous paired fins). Indeed, recent studies on the development of the lobed fin in N. forsteri have revealed the developmental mechanism of skeletal patterning (Johanson et al., 2004, 2007; Woltering et al., 2020). However, our current understanding of muscle and nerve development in lobed fins largely relies on descriptions and illustrations from classical literature (e.g., Semon, 1898; Salensky, 1899; Greil, 1913), which are insufficient for comparison with recent developmental studies. In this study, we seek to provide a basis for future developmental studies by describing the development of muscles in the pectoral lobed fin of N. forsteri based on three-dimensional observations at a cellular level.
Materials and Methods
Fertilized Neoceratodus forsteri eggs were legally collected from the Department of Biological Sciences, Macquarie University, Sydney, Australia, and transported with the permission of CITES (Certificate No. 2009-AU-564836) in 2009. The embryos and larvae were fixed with 10% neutral buffered formalin after Berlin blue dye injection to blood vessels by using a previously described method (Kamei et al., 2010), and stored in 10% neutral buffered formalin. All specimens are registered to the Iwate Medical University Ura Ryozi Collection (IMU-UR; Table 1). In this study, embryos were staged according to the Kemp (1982) stage table.
High-resolution X-ray tomography of the embryonic samples was performed at the synchrotron radiation facility SPring-8 in Sayo-cho, Hyogo Prefecture, Japan. The entire body of each embryo was placed in a polypropylene tube filled with normal saline in the experimental hutch of the beamline BL20B2 and scanned by means of propagation-based X-ray phase-contrast tomography (Paganin et al., 2002) with a voxel size of 2.70 μm at an energy of 15 keV. A visible-light conversion type X-ray image detector was used to detect X-ray transmission images. Incident X-ray image onto the detector was converted into visible light image by a Gadox scintillator with a thickness of 15 μm. Combinations of two camera lenses, 1st lens: a camera lens with a focal length of 35 mm (AI AF Nikkor 35 mm f/2D; Nikon, Tokyo, Japan); 2nd lens: a camera lens with a focal length of 85 mm (AI AF Nikkor 85 mm f/1.4D IF; Nikon, Tokyo, Japan), were used to form the image on a sCMOS image sensor (ORCA Flash C11440-22C; Hamamatsu Photonics, Hamamatsu, Japan). The distance from the sample to the X-ray image detector was 600 mm. A total of 1,800 projections covering 180° were taken with an exposure time of 200 ms per projection. Slices were reconstituted by using a filtered back-projection algorithm implemented on homemade software (Uesugi et al., 2010).
The stacks of images were examined by using the image-processing software package Fiji (Schindelin et al., 2012) in orthogonal views. The images were reconstructed three-dimensionally, using the Avizo software (Thermo Fisher Scientific, Waltham, MA, United States). Each embryonic component was manually labeled on the sections and subsequently combined to generate a three-dimensional model.
Results
Onset of the Pectoral Fin Bud
The earliest developmental stage we observed was stage 41 (Table 1). By this stage, the dorsal axial muscles have become differentiated, and the pronephros has developed at the caudal end of the pharynx. The prospective field of the pectoral fin bud, which is observable lateral to the pronephros, consists of the epidermis and mesenchyme, which together are as large as the pronephric duct as previously observed by Hodgkinson et al. (2009). At this stage, the mesenchyme, which later develops into ventral axial muscles and fin buds, does not extend ventrally to the pronephros.
At stage 46 (Figure 1 and Table 1), the pectoral fin bud protrudes laterally at the junction of the lateroventral aspect of the pronephros and the laterodorsal aspect of the ventral axial muscles. At this stage, the fin bud consists of the epidermis and mesenchyme, and the latter is homogeneous and structureless. Dorsal to the fin bud, there is little space between the epidermis and pronephros, and no mesenchymal tissue is observable. Positional relationships among the pectoral fin, pronephros, and ventral axial muscles are maintained throughout the observed developmental stages (stages 46–48).
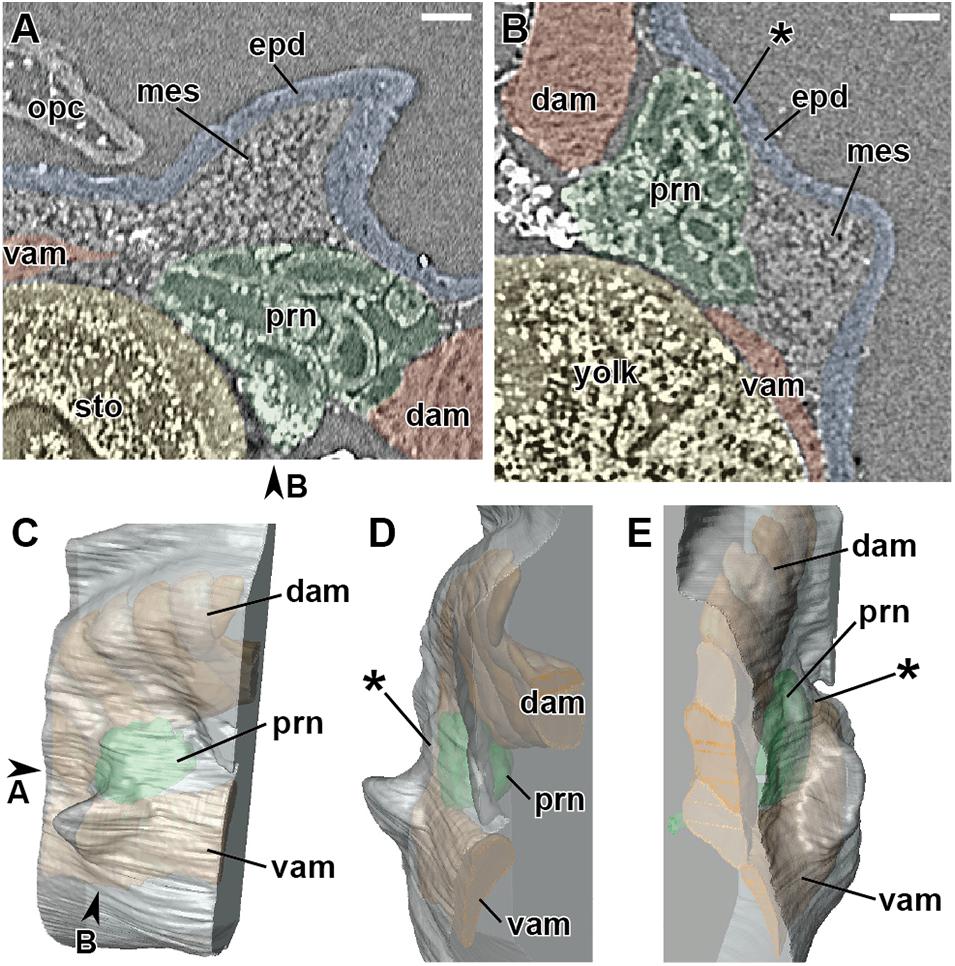
Figure 1. Developing right pectoral fin of Neoceratodus forsteri at stage 46 (total length = 13.5 mm). (A) Coronal section. (B) Transverse section. (C) Lateral view. (D) Cranial view. (E) Caudal view. dam, dorsal axial muscle; epd, epidermis; mes, mesenchyme; opc, operculum; prn, pronephros; sto, stomach; vam, ventral axial muscle. Arrowheads indicate the respective sectioned levels. The asterisk marks the junction between the axial body wall and fin bud, where no mesenchymal tissue is observable at this developmental stage. Scale bar = 100 μm.
Differentiation of the Pectoral Fin Muscles
Differentiation of the pectoral fin muscles occurs during stage 47. Here, we divide stage 47 into two phases (Table 1) based on when myotubes of the pectoral fin muscles become identifiable.
In the early phase of stage 47 (Figure 2), a mass of cartilage develops at the base of the pectoral fin. A dense mesenchymal cell mass (premuscle mass) surrounds the cartilage in the proximal part of the fin bud (Figures 2B–D). In the cranial part of the fin bud, a small number of mesenchymal cells (just five or six cells across at the thickest) occupy the space between the laterodorsal aspect of the pronephros and epidermis (Figure 2C). This mesenchyme becomes thinner caudally (Figure 2D), and in the caudal part, there is little space between them (Figure 2E).
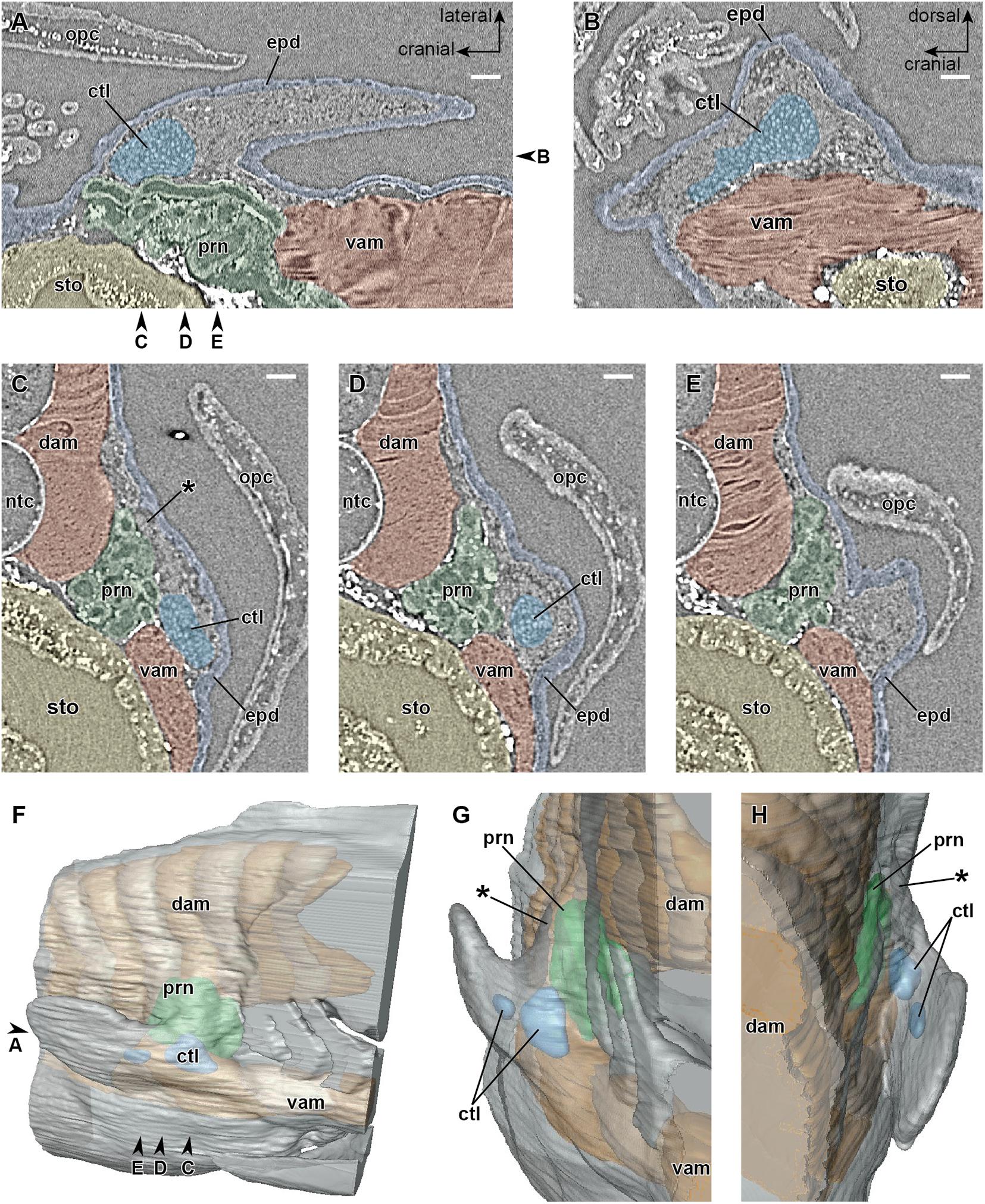
Figure 2. Developing right pectoral fin of Neoceratodus forsteri during the early phase of stage 47 (total length = 16.0 mm). (A) Coronal section. (B) Sagittal section. (C–E) Transverse section. (F) Lateral view. (G) Cranial view. (H) Caudal view. ctl, cartilage; dam, dorsal axial muscle; epd, epidermis; ntc, notochord; opc, operculum; prn, pronephros; sto, stomach; vam, ventral axial muscle. Arrowheads indicate the respective sectioned levels. The asterisk marks the cranial part of the junction between the axial body wall and fin bud, filled by a small number of mesenchymal cells at this developmental stage, which later form the cleithrum. Scale bar = 100 μm.
In the late phase of stage 47 (Figure 3), the primary myotubes of the pectoral fin adductor (Figures 3A,B) and abductor (Figures 3B,C) muscles become differentiated. The cartilage of the scapulocoracoid, humerus, and ulna are now recognizable separately (Figure 3B). It is identifiable that the adductor and abductor muscles are connected proximally to the scapulocoracoid and distally to the humerus and ulna, while separation of superficial and deep layers (superficiales and profunduses) of these muscles is not observable. At the cranial part of the junction between the pectoral fin and trunk, which corresponds approximately to the thickest part of the mesenchyme intervening between the pronephros and epidermis during the early phase of stage 47 (Figure 2C), the cleithrum develops as a dermal bone (Figures 3A–D) filling the space between the pronephros and epidermis (Figure 3D).
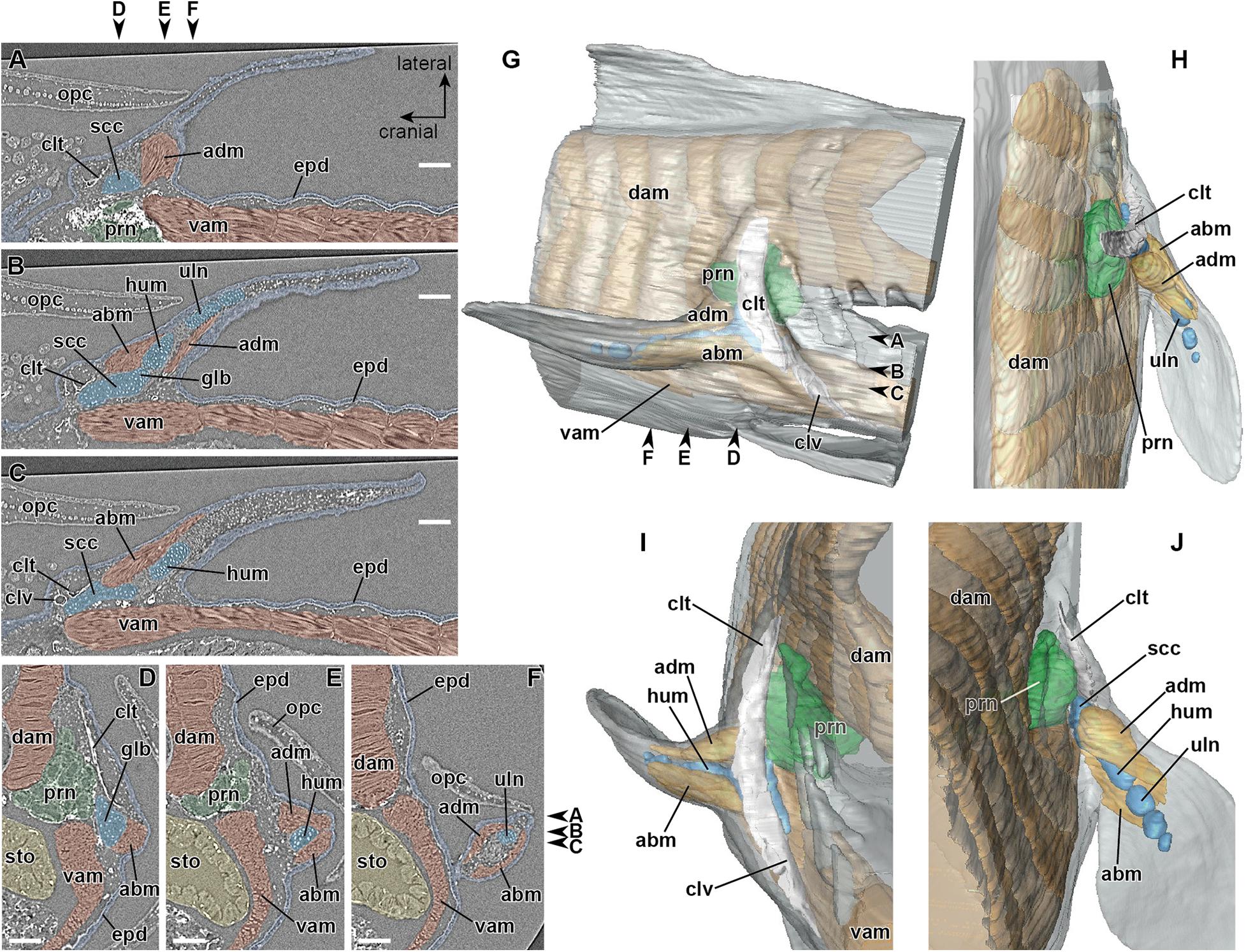
Figure 3. Developing right pectoral fin of Neoceratodus forsteri during the late phase of stage 47 (total length = 17.5 mm). (A–C) Coronal section. (D–F) Transverse section. (G) Lateral view. (H) Dorsal view. (I) Cranial view. (J) Caudal view. abm, abductor muscle; adm, adductor muscle; clt, cleithrum; clv, clavicle; dam, dorsal axial muscle; epd, epidermis; glb, glenoid buttress of the scapulocoracoid; hum, humerus; opc, operculum; prn, pronephros; scc, scapulocoracoid; sto, stomach; uln, ulna; vam, ventral axial muscle. Arrowheads indicate the respective sectioned levels. Scale bar = 200 μm.
Development of the Girdle Muscle
The axial skeleton develops from stage 47 onward. During the late phase of stage 47, the vertebral elements, namely the neural arch and basiventral arcualium, develop around the notochordal sheath. Subsequently, at the beginning of stage 48 (Table 1), the cranial rib extends toward the junction between the dorsal and ventral axial muscles at a level caudal to the pronephros. At this phase, no muscle tissue connected to the cleithrum is recognizable.
Later, in the mid-phase of stage 48 (Table 1), the girdle muscle, which corresponds to the retractor lateralis ventralis pectoralis (e.g., Diogo et al., 2016) and to the retractor cleithri muscle (Greil, 1913), becomes differentiated (Figure 4). This girdle muscle is connected proximally to the distal end of the cranial rib (Figures 4C,I), and distally to the medial surface of the cleithrum (Figures 4D,E,I). No connection of the girdle muscle to connective tissue associated with the axial muscles is observable.
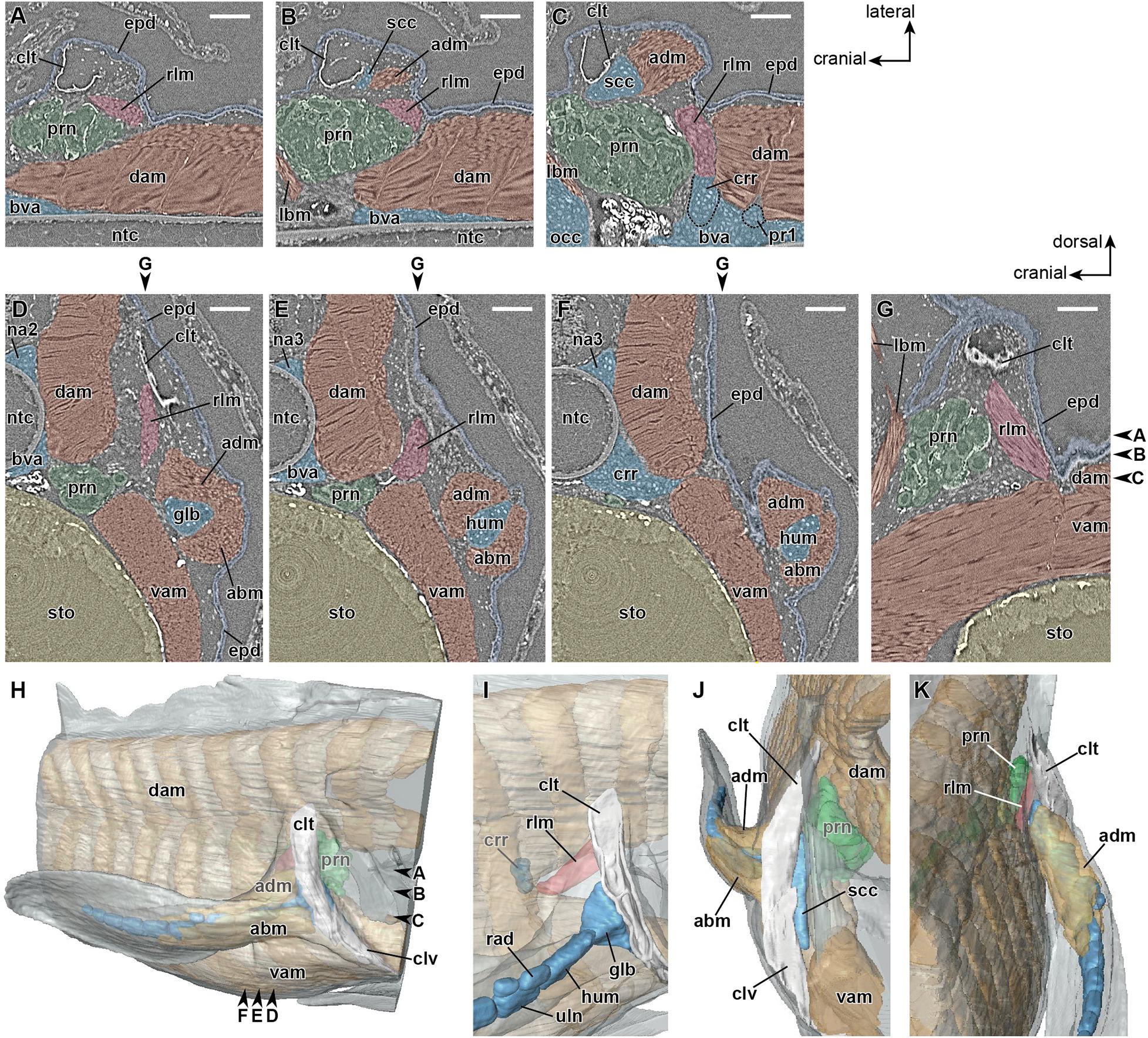
Figure 4. Developing right pectoral fin of Neoceratodus forsteri at stage 48 (total length = 20.5 mm). (A–C) Coronal section. (D–F) Transverse section. (G) Sagittal section. (H) Lateral view. (I) Enlarged image of the retractor laterralis ventralis pectoralis muscle in caudolateral view. (J) Cranial view. (K) Caudal view. abm, abductor muscle; adm, adductor muscle; bva, basiventral arcualium; clt, cleithrum; clv, clavicle; dam, dorsal axial muscle; crr, cranial rib; epd, epidermis; glb, glenoid buttress of the scapulocoracoid; lbm, levator arcuum branchialium five muscle (i.e., the dorsopharyngeus muscle in Greil, 1913); hum, humerus; na2, neural arch 2; na3, neural arch 3; ntc, notochord; opc, operculum; prn, pronephros; pr1, pleural rib 1; scc, scapulocoracoid; rad, radius; rlm, retractor lateralis ventralis pectoralis muscle (i.e., the retractor cleithri muscle in Greil, 1913); sto, stomach; uln, ulna; vam, ventral axial muscle. Arrowheads indicate the respective sectioned levels. Scale bar = 200 μm.
Subsequently, in the late phase of stage 48 (Table 1), the pectoral fin adductor and abductor muscles subdivide into superficial and deep layers (superficiales and profunduses), and the superficial layers of both muscles expand their proximal connections to the medial surface of the cleithrum.
Discussion
Developmental Environment for the Pectoral Fin Muscles and Their Innervating Nerves
In this study, we used synchrotron phase-contrast microtomography to perform histological observations of pectoral fin development in N. forsteri, in light of recent knowledge of vertebrate development. As the examined embryos and larvae possessed large yolk sacs and some mesenchymal tissues characterized by low cell densities, they were not suited for physical sectioning, which requires retention of heterogeneous tissues on slide glass. On the other hand, virtual sectioning by X-ray microtomography, as a non-destructive technique, can keep the morphologies of all tissues intact, and enables three-dimensional observation at a high resolution. Although resolutions are generally higher in physically prepared sections with staining, or the conventional histological technique, technological advances in X-ray microtomography will continue to provide indispensable contribution to comparative morphology. Future morphological and histological studies may request three-dimensional observations based on X-ray microtomography data. In addition, since the technique used in this study does not require any specific fixation nor chemical treatment, it will be useful to obtain three-dimensional data prior to other experiments, such as analyses on gene expressions.
Our results demonstrate that the pectoral fin of N. forsteri develops adjacent to the pronephros, and that there is only a small space available to accommodate the mesenchyme between the axial body wall and fin bud (Figures 1B, 2C–E). The minor mesenchyme intervening between the epidermis and pronephros at the cranial part of the fin bud base (asterisk in Figure 2C) later produces the dermal bone of the cleithrum (Figures 3D,H–J), thereby substantially narrowing the migratory routes of the somite-derived muscle progenitor cells and spinal nerve axons. Based on these observations, it is likely that the “plexus mesenchyme” (Wright and Snider, 1996) does not develop in N. forsteri. Among extant tetrapods, the mesenchyme occupying the space between the axial body wall and limb bud is similarly small during forelimb development in lissamphibians (Byrnes, 1898; Chen, 1935). In contrast to N. forsteri, however, the lack of a cleithrum in lissamphibians allows the mesenchymal environment to remain relatively large. Although further comparative analyses between N. forsteri and lissamphibians are needed, it is possible that the evolutionary reduction of the cleithrum in the basal tetrapodomorph lineage (Romer, 1924; Shubin et al., 2006; Molnar et al., 2017; Figure 5) allowed the establishment of a novel developmental environment, i.e., the “plexus mesenchyme.”
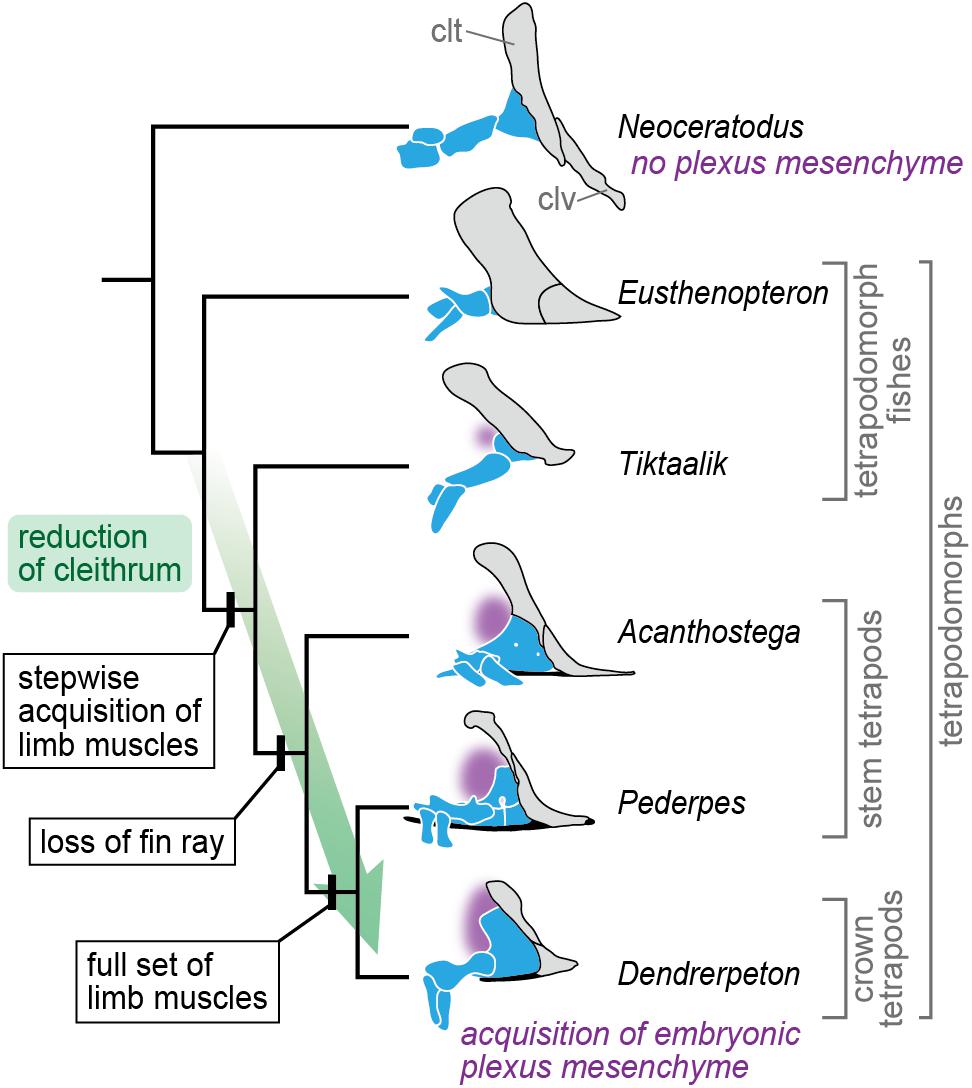
Figure 5. A scenario for evolution of the plexus mesenchyme. In the evolution leading to the crown tetrapods, the cleithrum gradually became reduced, and concurrently, the full set of limb muscles was acquired in a stepwise manner (Molnar et al., 2017). At this transitional phase, the brachial plexus might become evolutionarily fixed, being accompanied with an acquisition of the embryonic plexus mesenchyme, which replaced the cleithrum-forming embryonic domain. The cleithrum and clavicle are gray, endoskeletal shoulder girdle and limb skeleton blue, interclavicle black, and position of the plexus mesenchyme (in embryonic development) purple. Abbreviations: clt, cleithrum; clv, clavicle. The tetrapodomorph skeletons are redrawn from Andrews and Westoll (1970), Coates (1996), Clack and Finney (2005), Shubin et al. (2006), and Clack (2012).
Muscles Connected to the Cleithrum
Our observations roughly show the developmental processes of muscles connected to the cleithrum, including the girdle muscle (retractor lateralis ventralis pectoralis or retractor cleithri) and the superficial adductor and abductor muscles of the pectoral fin.
As the girdle muscle is connected proximally to the distal end of the rib and distally to the medial surface of the cleithrum, it is likely homologous with the tetrapod serratus muscle, which develops as an axial muscle (Valasek et al., 2010, 2011). We demonstrate that the girdle muscle in N. forsteri develops distinctly later than the dorsal and ventral axial muscles do (Figure 4), but could not identify its primordial cells probably due to rapid differentiation of the girdle muscle; similarly, the detailed developmental process of the tetrapod serratus muscle has also remained elusive (Pu et al., 2016). Nevertheless, in N. forsteri, the girdle muscle develops through either dorsocranial extension of the developing muscle from the junction between the dorsal and ventral axial muscles at the level of the cranial rib or differentiation of the mesenchyme occupying the space medial to the cleithrum, because the cleithrum and the axial muscles are separated at the corresponding developmental stage (Figures 3, 4).
In N. forsteri, the superficial layers of pectoral fin adductor and abductor muscles, which are homologous with the latissimus dorsi and pectoralis muscles, respectively, become differentiated substantially later than do the deep layers (Figure 3). In limb muscle development, these superficial muscles (latissimus dorsi and pectoralis) develop through an “in-out” process, in which the muscle progenitor cells, upon entering the limb bud, extend out from the limb bud onto the axial body wall (Valasek et al., 2011). Based on our observations, in N. forsteri, it is probable that the superficial adductor and abductor muscles develop not through the “in-out” process, as the fin bud is occupied by myotubes of the deep adductor and abductor muscles and includes very few undifferentiated muscle progenitor cells (Figure 3). This suggests that the “in-out” developmental process of superficial limb muscles first evolved in the tetrapodomorph lineage.
Perspectives and Conclusion
The tetrapod limb is an evolutionary novelty: new muscles were acquired in the early evolution of limbed tetrapods (Molnar et al., 2017, 2018), and a new variational modality of neuromuscular morphology was established during the fin-to-limb transition (Hirasawa and Kuratani, 2018). Soft-tissue anatomy of fossil vertebrates can often be reconstructed by using phylogenetic bracketing approach (Witmer, 1995), but such approach is ineffective for inferring a transitional state prior to an origin of an evolutionary novelty. Therefore, the process of the soft-tissue evolution during the fin-to-limb transition cannot be deciphered solely through comparative morphological analyses between extant and fossil taxa, although these analyses, together with biomechanics, potentially contribute to an understanding of functional transitions independent of the origin of the evolutionary novelty. Instead, like the vertebrate jaw, another example of evolutionary novelty (Shigetani et al., 2002), the origin of the novel limb soft-tissue patterns will be elucidated by analyzes of developmental genetic bases.
In this study, we conducted three-dimensional observations of the pectoral lobed fin of N. forsteri in light of recent knowledge of vertebrate development, by using synchrotron radiation X-ray microtomography. We found that the mesenchyme occupying the junction between the axial body wall and fin bud is quite small in N. forsteri, implying the absence of the “plexus mesenchyme” seen in tetrapods. In addition, our observations highlighted the late differentiation timings of the girdle muscle and superficial adductor and abductor fin muscles separate from those of the other axial muscles and deep adductor and abductor fin muscles, respectively. These characteristics of pectoral lobed fin development in N. forsteri will lead to a better understanding of the fin-to-limb transition from the viewpoint of evolutionary developmental biology.
Data Availability Statement
The raw data supporting the conclusions of this article will be made available by the authors, without undue reservation.
Ethics Statement
Ethical review and approval was not required for the animal study because fish species are beyond the scope of ethical review in Japan. However, we conducted the research under self-assessment of ethics.
Author Contributions
TH conceived the project. SI collected and prepared the embryonic samples. TH and CC wrote the draft. All authors read and approved the final version of the manuscript and together conducted the synchrotron radiation X-ray tomography of the embryonic samples.
Funding
This work was supported by JSPS KAKENHI grant nos. 17H06385 and 19K04061 (to TH), Coordenação de Aperfeiçoamento de Pessoal de Nível Superior, Brasil (CAPES) Finance Code 001-Programa Nacional de Pós Doutorado and UERJ Programa de Apoio à Docência (PAPD) process number E-26/007/10661/2019 (to CC), and CNPq #310101/2017-4 and FAPERJ #E-26/202.890/2018 grants (to PMB).
Conflict of Interest
The authors declare that the research was conducted in the absence of any commercial or financial relationships that could be construed as a potential conflict of interest.
Acknowledgments
We thank Jean Joss (Macquarie University) for providing Neoceratodus embryos, and Iwate Medical University for their management of the collection of all specimens used in this study. We also appreciate two referees for providing useful comments, which definitely improved our manuscript.
References
Ahlberg, P. E. (2019). Follow the footprints and mind the gaps: a new look at the origin of tetrapods. Earth Environ. Sci. Trans. R. Soc. Edinb. 109, 115–137. doi: 10.1017/s1755691018000695
Amemiya, C. T., Alfoldi, J., Lee, A. P., Fan, S. H., Philippe, H., MacCallum, I., et al. (2013). The African coelacanth genome provides insights into tetrapod evolution. Nature 496, 311–316.
Andrews, S. M., and Westoll, T. S. (1970). The postcranial skeleton of Eusthenopteron foodi whiteaves. Trans. R. Soc. Edinb. 68, 207–329. doi: 10.1017/s008045680001471x
Betancur-R, R., Wiley, E. O., Arratia, G., Acero, A., Bailly, N., Miya, M., et al. (2017). Phylogenetic classification of bony fishes. BMC Evol. Biol. 17:162. doi: 10.1186/s12862-017-0958-3
Braus, H. (1901). Die muskeln und nerven der ceratodusflosse. Ein beitrag zur vergleichenden morphologie der freien gliedmaasse bei niederen fischen und zur archipterygiumtheorie. Denkschr. Med. Naturwiss. Ges. Jena 4, 137–300.
Byrnes, E. F. (1898). Experimental studies on the development of limb muscles in amphibia. J. Morphol. 14, 105–140. doi: 10.1002/jmor.1050140202
Chen, H. K. (1935). Development of the pectoral limb of Necturus maculosus. Illinois Biol. Monogr. 14, 1–71. doi: 10.2307/1437018
Clack, J. A. (2012). Gaining Ground: The Origin and Evolution of Tetrapods, 2nd Edn. Bloomington: Indiana University Press.
Clack, J. A., and Finney, S. M. (2005). Pederpes finneyae, an articulated tetrapod from the Tournaisian of Western Scotland. J. Syst. Palaeontol. 2, 311–346. doi: 10.1017/s1477201904001506
Cloutier, R., Clement, A. M., Lee, M. S. Y., Noel, R., Bechard, I., Roy, V., et al. (2020). Elpistostege and the origin of the vertebrate hand. Nature 579, 549–554. doi: 10.1038/s41586-020-2100-8
Coates, M. I. (1996). The devonian tetrapod Acanthostega gunnari jarvik: postcranial anatomy, basal tetrapod interrelationships and patterns of skeletal evolution. Trans. R. Soc. Edinb. Earth Sci. 87, 363–421. doi: 10.1017/s0263593300006787
Davis, M. C., Dahn, R. D., and Shubin, N. H. (2007). An autopodial-like pattern of Hox expression in the fins of a basal actinopterygian fish. Nature 447, 473–476. doi: 10.1038/nature05838
Dietrich, S., Schubert, F. R., Healy, C., Sharpe, P. T., and Lumsden, A. (1998). Specification of the hypaxial musculature. Development 125, 2235–2249. doi: 10.1242/dev.125.12.2235
Diogo, R., Johnston, P., Molnar, J. L., and Esteve-Altava, B. (2016). Characteristic tetrapod musculoskeletal limb phenotype emerged more than 400 MYA in basal lobe-finned fishes. Sci. Rep. 6:37592.
Fürbringer, M. (1888). Untersuchungen zur Morphologie und Systematik der Vögel: zugleich ein Beitrag zur Anatomie der Stütz- und Bewegungsorgane. Amsterdam: Van Holkema, 1751.
Grandel, H., and Schulte-Merker, S. (1998). The development of the paired fins in the zebrafish (Danio rerio). Mech. Dev. 79, 99–120. doi: 10.1016/s0925-4773(98)00176-2
Greil, A. (1913). Entwickelungsgeschichte des Kopfes und des Blutgefäßsystems von Ceratodus forsteri. II. Die epigenetischen Erwerbungen während der Stadien 39-48. Denkschr. Med. Naturwiss. Ges. Jena 4, 935–1492.
Gross, M. K., Moran-Rivard, L., Velasquez, T., Nakatsu, M. N., Jagla, K., and Goulding, M. (2000). Lbx1 is required for muscle precursor migration along a lateral pathway into the limb. Development 127, 413–424. doi: 10.1242/dev.127.2.413
Haase, G., Dessaud, E., Garcès, A., de Bovis, B., Birling, M. C., Filippi, P., et al. (2002). GDNF acts through PEA3 to regulate cell body positioning and muscle innervation of specific motor neuron pools. Neuron 35, 893–905. doi: 10.1016/s0896-6273(02)00864-4
Hirasawa, T., and Kuratani, S. (2018). Evolution of the muscular system in tetrapod limbs. Zoolog. Lett. 4:27.
Hodgkinson, V. S., Ericsson, R., Johanson, Z., and Joss, J. M. P. (2009). The apical ectodermal ridge in the pectoral fin of the Australian lungfish (Neoceratodus forsteri): keeping the fin to limb transition in the fold. Acta Zool. 90, 253–263. doi: 10.1111/j.1463-6395.2008.00349.x
Johanson, Z., Joss, J., Boisvert, C. A., Ericsson, R., Sutija, M., and Ahlberg, P. E. (2007). Fish fingers: digit homologues in sarcopterygian fish fins. J. Exp. Zool. B Mol. Dev. Evol. 308, 757–768. doi: 10.1002/jez.b.21197
Johanson, Z., Joss, J. M. P., and Wood, D. (2004). The scapulocoracoid of the queensland lungfish Neoceratodus forsteri (Dipnoi : Sarcopterygii): morphology, development and evolutionary implications for bony fishes (Osteichthyes). Zoology 107, 93–109. doi: 10.1016/j.zool.2004.01.001
Jung, H., Baek, M., D’Elia, K. P., Boisvert, C., Currie, P. D., Tay, B. H., et al. (2018). The ancient origins of neural substrates for land walking. Cell 172, 667–682. doi: 10.1016/j.cell.2018.01.013
Kamei, M., Isogai, S., Pan, W., and Weinstein, B. M. (2010). Imaging blood vessels in the zebrafish. Methods Cell Biol. 100, 27–54. doi: 10.1016/b978-0-12-384892-5.00002-5
Kemp, A. (1982). The embryological development of the queensland lungfish, Neoceratodus forsteri (Krefft). Mem. Queensl. Mus. 20, 553–597.
Kramer, E. R., Knott, L., Su, F. Y., Dessaud, E., Krull, C. E., Helmbacher, F., et al. (2006). Cooperation between GDNF/Ret and ephrinA/EphA4 signals for motor-axon pathway selection in the limb. Neuron 50, 35–47. doi: 10.1016/j.neuron.2006.02.020
Miyake, T., Kumamoto, M., Iwata, M., Sato, R., Okabe, M., Koie, H., et al. (2016). The pectoral fin muscles of the coelacanth Latimeria chalumnae: functional and evolutionary implications for the fin-to-limb transition and subsequent evolution of tetrapods. Anat. Rec. 299, 1203–1223. doi: 10.1002/ar.23392
Mollier, S. (1897). Die paarigen extremitäten der wirbeltiere. III. Die entwickelung der paarigen flossen des stöhrs. Anat. Hefte 8, 1–74. doi: 10.1007/bf02316963
Molnar, J. L., Diogo, R., Hutchinson, J. R., and Pierce, S. E. (2017). Reconstructing pectoral appendicular muscle anatomy in fossil fish and tetrapods over the fins-to-limbs transition. Biol. Rev. 93, 1077–1107. doi: 10.1111/brv.12386
Molnar, J. L., Diogo, R., Hutchinson, J. R., and Pierce, S. E. (2018). Evolution of hindlimb muscle anatomy across the tetrapod water-to-land transition, including comparisons with forelimb anatomy. Anat. Rec. 303, 218–234. doi: 10.1002/ar.23997
Murakami, Y., and Tanaka, M. (2011). Evolution of motor innervation to vertebrate fins and limbs. Dev. Biol. 355, 164–172. doi: 10.1016/j.ydbio.2011.04.009
Nakamura, T., Gehrke, A. R., Lemberg, J., Szymaszek, J., and Shubin, N. H. (2016). Digits and fin rays share common developmental histories. Nature 536, 225–228. doi: 10.1038/nature19322
Okamoto, E., Kusakabe, R., Kuraku, S., Hyodo, S., Robert-Moreno, A., Onimaru, K., et al. (2017). Migratory appendicular muscles precursor cells in the common ancestor to all vertebrates. Nat. Ecol. Evol. 1, 1731–1736. doi: 10.1038/s41559-017-0330-4
Paganin, D., Mayo, S. C., Gureyev, T. E., Miller, P. R., and Wilkins, S. W. (2002). Simultaneous phase and amplitude extraction from a single defocused image of a homogeneous object. J. Microsc. 206, 33–40. doi: 10.1046/j.1365-2818.2002.01010.x
Pierce, S. E., Clack, J. A., and Hutchinson, J. R. (2012). Three-dimensional limb joint mobility in the early tetrapod Ichthyostega. Nature 486, 523–526. doi: 10.1038/nature11124
Pu, Q., Huang, R., and Brand-Saberi, B. (2016). Development of the shoulder girdle musculature. Dev. Dyn. 245, 342–350. doi: 10.1002/dvdy.24378
Romer, A. S. (1924). Pectoral limb musculature and shoulder-girdle structure in fish and tetrapods. Anat. Rec. 27, 119–143. doi: 10.1002/ar.1090270210
Saito, H. (1988a). Development of the vasculature of the pectoral fin in the Australian lungfish, Neoceratodus forsteri. Am. J. Anat. 182, 183–196. doi: 10.1002/aja.1001820208
Saito, H. (1988b). The development of the vasculature of the pelvic fin in the Australian lungfish, Neoceratodus forsteri. Tohoku J. Exp. Med. 156, 359–373. doi: 10.1620/tjem.156.359
Salensky, W. (1899). “Entwicklungsgeschichte des ichthyopterygiums,” in Proceedings of the 4th International Congress of Zoology, Cambridge, 177–183.
Sanchez, S., Dupret, V., Tafforeau, P., Trinajstic, K. M., Ryll, B., Gouttenoire, P. J., et al. (2013). 3D microstructural architecture of muscle attachments in extant and fossil vertebrates revealed by synchrotron microtomography. PLoS One 8:e56992. doi: 10.1371/journal.pone.0056992
Schindelin, J., Arganda-Carreras, I., Frise, E., Kaynig, V., Longair, M., Pietzsch, T., et al. (2012). Fiji: an open-source platform for biological-image analysis. Nat. Methods 9, 676–682. doi: 10.1038/nmeth.2019
Semon, R. (1898). Die entwickelung der paarigen flossen des Ceratodus forsteri. Denkschr. Med. Naturwiss. Ges. Jena 4, 59–111.
Shann, E. W. (1920). The comparative myology of the shoulder girdle and pectoral fin of fishes. Trans. R. Soc. Edinb. 52, 531–570. doi: 10.1017/s0080456800004452
Shann, E. W. (1924). Further observations on the myology of the pectoral region in fishes. Proc. Zool. Soc. Lond. 94, 195–215. doi: 10.1111/j.1096-3642.1924.tb01497.x
Shigetani, Y., Sugahara, F., Kawakami, Y., Murakami, Y., Hirano, S., and Kuratani, S. (2002). Heterotopic shift of epithelial-mesenchymal interactions in vertebrate jaw evolution. Science 296, 1316–1319. doi: 10.1126/science.1068310
Shubin, N. H., and Alberch, P. (1986). A morphogenetic approach to the origin and basic organization of the tetrapod limb. Evol. Biol. 20, 319–387. doi: 10.1007/978-1-4615-6983-1_6
Shubin, N. H., Daeschler, E. B., and Coates, M. I. (2004). The early evolution of the tetrapod humerus. Science 304, 90–93. doi: 10.1126/science.1094295
Shubin, N. H., Daeschler, E. B., and Jenkins, F. A. (2006). The pectoral fin of Tiktaalik roseae and the origin of the tetrapod limb. Nature 440, 764–771. doi: 10.1038/nature04637
Tanaka, M. (2016). Fins into limbs: autopod acquisition and anterior elements reduction by modifying gene networks involving 5’Hox, Gli3, and Shh. Dev. Biol. 413, 1–7. doi: 10.1016/j.ydbio.2016.03.007
Turner, N., Mikalauskaite, D., Barone, K., Flaherty, K., Senevirathne, G., Adachi, N., et al. (2019). The evolutionary origins and diversity of the neuromuscular system of paired appendages in batoids. Proc. R. Soc. B Biol. Sci. 286:20191571. doi: 10.1098/rspb.2019.1571
Uesugi, K., Hoshino, M., Takeuchi, A., Suzuki, Y., Yagi, N., and Nakano, T. (2010). Development of fast (sub-minute) micro-tomography. AIP Conf. Proc. 1266, 47–50.
Ura, R. (1956). Kontribuo al ontogenio de vazsistemo de antauaj membroj, precipe ce Hymobius naevius: unu provo solvi membroproblemon. Okajimas Folia Anat. Jpn. 28, 256–285.
Valasek, P., Theis, S., DeLaurier, A., Hinits, Y., Luke, G. N., Otto, A. M., et al. (2011). Cellular and molecular investigations into the development of the pectoral girdle. Dev. Biol. 357, 108–116. doi: 10.1016/j.ydbio.2011.06.031
Valasek, P., Theis, S., Krejci, E., Grim, M., Maina, F., Shwartz, Y., et al. (2010). Somitic origin of the medial border of the mammalian scapula and its homology to the avian scapula blade. J. Anat. 216, 482–488. doi: 10.1111/j.1469-7580.2009.01200.x
Wagner, G. P. (2014). Homology, Genes, and Evolutionary Innovation. Princeton, NY: Princeton University Press, 496.
Witmer, L. M. (1995). “The extant phylogenetic bracket and the importance of reconstructing soft tissue in fossils,” in Functional Morphology in Vertebrate Paleontology, ed. J. J. Thomason (Cambridge: Cambridge University Press), 19–33.
Woltering, J. M., Irisarri, I., Ericsson, R., Joss, J. M. P., Sordino, P., and Meyer, A. (2020). Sarcopterygian fin ontogeny elucidates the origin of hands wiith digits. Sci. Adv. 6:eabc3510. doi: 10.1126/sciadv.abc3510
Wright, D. E., and Snider, W. D. (1996). Focal expression of glial cell line-derived neurotrophic factor in developing mouse limb bud. Cell Tissue Res. 286, 209–217. doi: 10.1007/s004410050689
Wynd, B. M., Daeschler, E. B., and Stocker, M. R. (2019). Evolutionary homology in the fin-to-limb transition: evaluating the morphology of foramina in a late devonian humerus from the catskill formation, Clinton County, Pennsylvania. J. Vertebr. Paleontol. 39:e1718682. doi: 10.1080/02724634.2019.1718682
Keywords: Sarcopterygii, Dipnomorpha, Tetrapodomorpha, fish-tetrapod transition, migratory muscle precursor cell, limb muscle, brachial plexus
Citation: Hirasawa T, Cupello C, Brito PM, Yabumoto Y, Isogai S, Hoshino M and Uesugi K (2021) Development of the Pectoral Lobed Fin in the Australian Lungfish Neoceratodus forsteri. Front. Ecol. Evol. 9:679633. doi: 10.3389/fevo.2021.679633
Received: 12 March 2021; Accepted: 01 July 2021;
Published: 22 July 2021.
Edited by:
Catherine Anne Boisvert, Curtin University, AustraliaReviewed by:
Koh Onimaru, RIKEN Center for Life Science Technologies, JapanZerina Johanson, Natural History Museum, United Kingdom
Copyright © 2021 Hirasawa, Cupello, Brito, Yabumoto, Isogai, Hoshino and Uesugi. This is an open-access article distributed under the terms of the Creative Commons Attribution License (CC BY). The use, distribution or reproduction in other forums is permitted, provided the original author(s) and the copyright owner(s) are credited and that the original publication in this journal is cited, in accordance with accepted academic practice. No use, distribution or reproduction is permitted which does not comply with these terms.
*Correspondence: Tatsuya Hirasawa, hirasawa@eps.s.u-tokyo.ac.jp