Beam Splitters Fabricated by Nonlinear Focusing of Femtosecond Laser Writing in Pure YAG Crystal
- 1Tianjin Key Laboratory of Micro-scale Optical Information Science and Technology, Insititute of Modern Optics, Nankai University, Tianjin, China
- 2Tianjin Key Laboratory of Optoelectronic Sensor and Sensing Network Technology, Tianjin, China
- 3Shandong Provincial Engineering and Technical Center of Light Manipulations & Shandong Provincial Key Laboratory of Optics and Photonic Device, School of Physics and Electronics, Shandong Normal University, Jinan, China
We report on the fabrication of waveguides and beam splitters in pure YAG crystals by femtosecond laser direct writing (FLDW). During the femtosecond laser writing process, a positive refractive index is induced through the nonlinear focusing above the focus position, resulting in an unusual guiding cross-sectional configuration. The supported guiding modes at 632.8 nm are measured and analyzed using the end–face coupling system. The propagation loss can be as low as 1.9 dB/cm for the single-line waveguide. Different from the geometry of the traditional fs-laser modified area, this novel structure might offer a new approach in the quest toward integrated photonics.
Introduction
Optical waveguides, as the basic component of integrated photonics, can confine the propagation of light within small volumes and play a non-negligible role in optical communication and optical information processing. FLDW has emerged to become an effective and mature piece of technology for optical waveguide fabrication over decades of fast development [1]. Compared with the other traditional waveguide fabrication techniques, such as ion implantation and reactive ion etching, FLDW has the unique features of flexible 3D fabrication configurations, good compatibility with a wide range of materials, and simplicity in use [2–4]. A femtosecond laser (fs-laser) will induce multiphoton absorption when modifying material properties and tailoring microstructures inside the transparent bulk materials with a much shorter timescale. Therefore, the thermal energy exchange between photo-excited electrons and lattice ions will be curbed. A smooth photo-modified region with extremely high spatial resolution can be achieved.
Due to the complex interaction between the fs-laser pulses and the materials, the modified guiding structures in glass, crystalline materials, and ceramics have greater diversity [5]. Normally, the structural types can be categorized as single-line waveguide with positive refractive index changes induced at the focused area, stress-field–induced double-line waveguides based on damage and negative refractive index changes generated at the focused area, and depressed-cladding waveguide formed by the combination of small tracks with negative refractive index change [5, 6]. Until now, those aforementioned guiding structures fabricated by FLDW have been demonstrated in a handful of host materials, including silica glass, ZBLAN, LiNbO3, KTN, YAG, YCOB, etc. [7–13]. It is beyond doubt that the designable prototypes of FLDW enable us make significant breakthroughs, from scientific research to technological devices, for example, beam splitters, optical couplers, MZI EO modulators, waveguide lasers, waveguide-based frequency converters, and topological fractal insulators [14–23]. Among these devices, optical waveguide power splitters, which can switch the optical signal from single input to multiple outputs, act as essential elements for integrated photonic circuits.
In this work, we report on the formation of new single-line waveguides and a beam splitter (1 × 3) in pure YAG crystals by FLDW. Pure YAG crystals are one of the most significant optical window materials, with high mechanical strength and stable physical and chemical properties. Meanwhile, the most distinctive feature of the obtained structures in the material is that the guiding area does not exist at the focused area but is posited at the area formed by the self-focus of the pulse lasers. The guiding properties of the guiding structures have been investigated. It is noted that both of the single-line waveguides and the beam splitter support fundamental-mode laser propagating in the structures at 632.8 nm with polarization-insensitive properties.
Experiments
An amplified Ti:sapphire laser system (Astrella, Coherent, United States), which delivered linearly polarized pulses of 40 fs and a central wavelength of 800 nm at a repetition rate of 1 kHz, was utilized to fabricate single-line waveguides and a beam splitter in the pure YAG crystal with dimensions of 20 × 10 × 2 mm3. During the fabricating process, the pure YAG sample was placed at a computer-controlled XYZ translation stage, and the fs-laser beam was focused using a 25 × microscope objective (N.A. = 0.4) at a depth of 100 μm beneath one of the 20 × 10-mm2 surfaces. As shown in Figure 1, three single-line waveguides (WG1, WG2, and WG3, 20 mm in length) and a waveguide beam splitter (WG4 with configurations of 1 × 3 splitters) were fabricated. The structure of the beam splitter was designed as follows: the length of every straight waveguide is 2 mm, the lengths of every two splitting waveguides are 7 mm and the splitting angle is 0.8°, the straight input arm was split into two branches and four branches, and finally, three straight waveguides are output because two of them were combined into one. These guiding configurations were fabricated with different pulse energies and scanning speeds. The micromachining parameters are listed in Table 1.
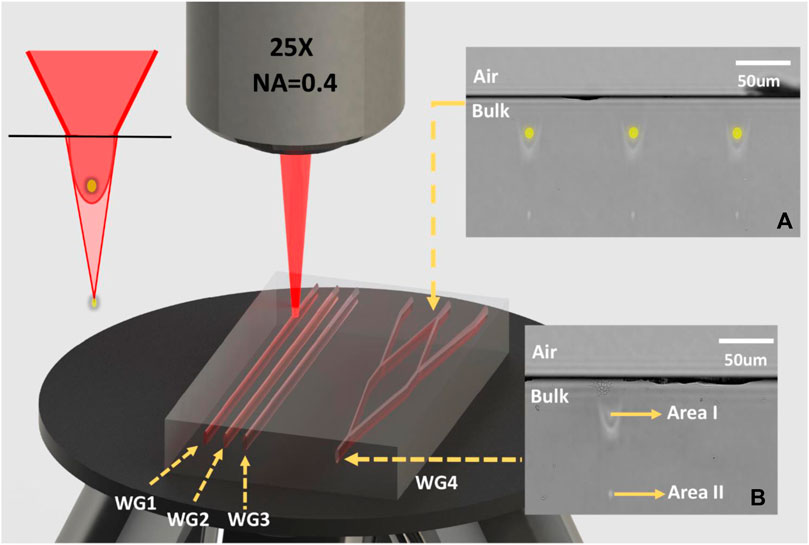
FIGURE 1. Schematic diagram of the laser writing single-line waveguides (WG1–WG3) and the beam splitter (WG4) in the pure YAG crystal. The insets are the microscope images of the input face (B) and the output face (A) of the waveguide beam splitter WG4 in the pure YAG crystal.
To further explore the guiding properties of the fabricated structures, we used an end–face coupling system to measure guiding-mode profiles at 632.8 nm, excited from the He–Ne laser. A Glan–Taylor prism and a half-wave plate were employed together to control the polarization of the incident laser beams. The laser is then focused to the input face of the waveguide after passing through a microscope objective (40 ×, N.A. = 0.65). Another microscope objective with a long working distance (50×, N.A. = 0.42) is used to collect the light field from the exit end face of the waveguide. The modal profiles are imaged using a CCD camera (WCD-UCD 12-1310, DataRay, United States), and the output intensities are measured using a power meter.
Results and Discussion
Figure 1 depicts a brief diagram of beam propagation. The insets are microscopic images of the input face and the output face of the waveguide beam splitter (WG4). In Figure 1B, we found that the induced track shows relatively complicated structures compared with the traditional modified region. The upper region (Area I) was formed by the self-focus of the pulse. The lower region (Area II) with local minimum area was formed by the laser focus. Both regions expand elliptically. Area I expands elliptically and is identified as the guiding area, which is confirmed through the microscope observation and the end–face coupling research. In a manner of speaking, the guiding volume is surrounded by irregular regions with a low refractive index, where the fs-laser intensity is higher than the ionization threshold of the material. As shown in the schematic diagram of the FLDW processing, the size of WG1–WG3 increases in order due to the gradual changes in the processing parameters.
Figure 2 displays the 2D and 3D modal profiles of the output light field collected from WG1, WG2, and WG3. We can see that the modal profiles exhibit fundamental modes at TM polarization and TE polarization, which is beneficial for excitation and coupling, reducing losses, and avoiding signal distortion. Meanwhile, the beam splitter WG4 supports the laser propagating at both TM and TE polarizations. It can be identified that these guiding structures perform insensitive propagating properties to the polarization of the laser. Figure 3A depicts the experimental, measured 2D and 3D guiding-mode profiles of the WG4 at TM polarization. Although the branch configurations are introduced to the design, the guiding mode still exhibited the fundamental-mode feature, which demonstrates the opportunity for this structure to contribute to the photonics circuits. The measured output intensity–splitting ratio for the three arms is about 1:1.84:1, which is very close to the simulated result (i.e., 1:1.95:1). Moreover, we can optimize the structure design and processing parameters to improve the splitting ratio. Figure 3B shows the simulated results of the 2D and 3D guiding-mode profiles. By comparing the experimental and simulated laser profiles, one can come to the conclusion that the experiment data match quite well with the simulated results. We believe that it is possible to achieve an adjustable beam-splitting ratio through improved structural design.
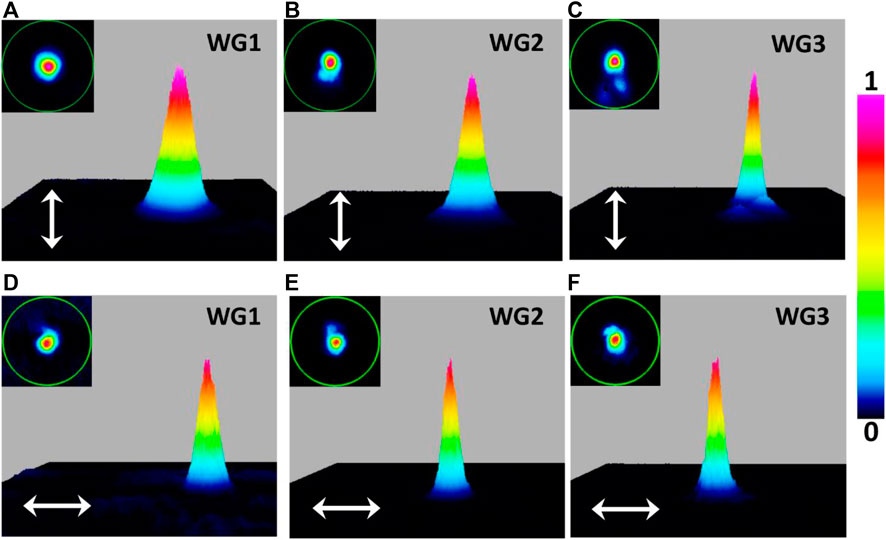
FIGURE 2. The 2D and 3D modal profiles of the output light field of the single-line waveguides WG1 at (A) TM polarization, (D) TE polarization, WG2 at (B) TM polarization, (E) TE polarization, and WG3 at (C) TM polarization, (F) TE polarization.
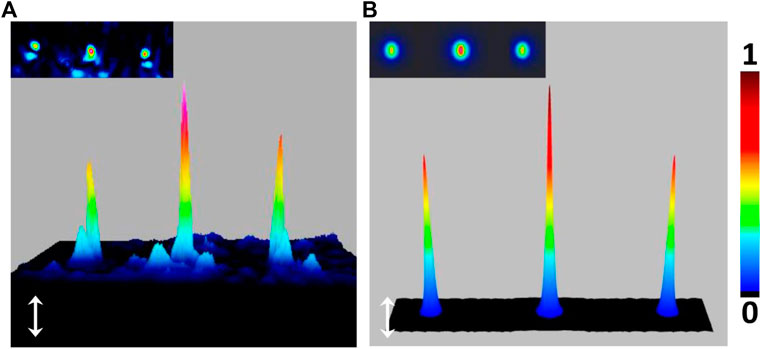
FIGURE 3. (A) Experimental results of 2D and 3D guiding-mode profiles of the beam splitter WG4 at TM polarization. (B) Simulated results of 2D and 3D guiding-mode profiles of the beam splitter WG4 at TM polarization.
Here, BeamPROP (Rsoft, Inc.) software based on the finite-difference beam propagation method (FD-BPM) [24] is used to simulate the light propagation at 632.8 nm in WG4. The top view of the simulated light intensity profile after light propagating on the XY-plane at TM polarization can be seen in Figure 4A. Figure 4B depicts the simulated evolution process of light propagating; clearly, the input light beam was split into three branches and still transmitted as fundamental modes. Insignificant light leakage can be observed at the junction points, which is mainly caused by structural imperfection and will increase the total losses to a certain extent.
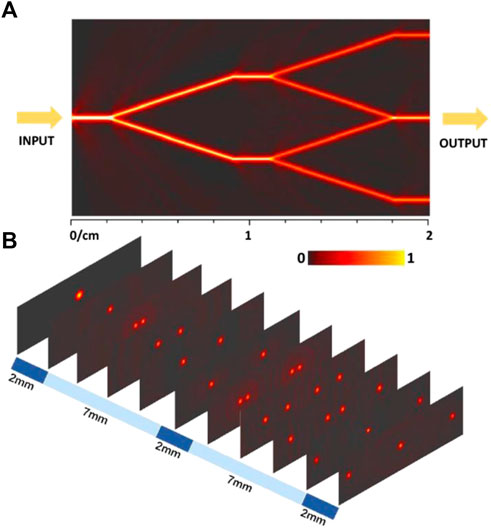
FIGURE 4. (A) Top view of the simulated light intensity profile after light propagating on the XY-plane at 632.8 nm at TM polarization. (B) Evolution process of light propagating.
Table 1 reveals the losses of the waveguides at 632.8 nm when the incident laser is with different polarizations. The total losses α are measured using the power meter and the end–face coupling system using the following formula:
where Pin and Pout correspond to the input and output laser powers, and the coupling losses are roughly calculated using the BeamPROP module of Rsoft Photonics CADSuite. By subtracting the coupling loss from the total loss for each polarization component, we can calculate the propagating loss. According to the calculated results, the difference in coupling losses is mainly related to the dimensions of these waveguides. Also, the propagating losses at TE polarization are slightly larger than those at TM polarization. The reason for this is that the geometries of the fabricated guiding structures are not centrosymmetric. The width along TM polarization is a little larger than that along TE polarization. The propagation loss can be as low as 1.9 dB/cm for the single-line waveguide WG1. Comparing the propagation losses of the single-line waveguide WG1 and the beam splitter WG4 with the same fabrication parameters, the additional losses may be partly attributed to the radiation loss caused by beam deflection and the mode coupling between the straight waveguides and the branch waveguide. For the splitter waveguide, the propagation losses in these two polarizations are roughly the same, which may be related to the structural design of the beam splitter.
Conclusion
In summary, we have demonstrated the fabrication of a waveguide beam splitter in a pure YAG crystal by femtosecond laser direct writing. For the waveguides and the beam splitter with positive refractive index changes, fundamental-mode guidance is achieved along both TE and TM polarizations at the wavelength of 632.8 nm. The experimentally measured modal profiles of the waveguide beam splitter are in good agreement with the simulation results. The research shows that the excellent beam-splitting performance of the structure is helpful for us to split beams in a certain proportion, which implies that our fabrication on the waveguide beam splitter has a potential application in integrated photonics.
Data Availability Statement
The original contributions presented in the study are included in the article/Supplementary Material; further inquiries can be directed to the corresponding author.
Author Contributions
HL and PW proposed the original idea and supervised the project. SY performed the experiments and measurements and was responsible for writing the manuscript. YR supported the formal analysis. All authors contributed to the article and approved the submitted version.
Funding
This work is supported by the National Natural Science Foundation of China (NSFC) (Grants no. 61575097 and 11704201), the National Natural Science Foundation of Tianjin City (NSFTJ) (17JCQNJC01600 and 19JCZDJC32700), and the Fundamental Research Funds for the Central Universities.
Conflict of Interest
The authors declare that the research was conducted in the absence of any commercial or financial relationships that could be construed as a potential conflict of interest.
Publisher’s Note
All claims expressed in this article are solely those of the authors and do not necessarily represent those of their affiliated organizations, or those of the publisher, the editors and the reviewers. Any product that may be evaluated in this article, or claim that may be made by its manufacturer, is not guaranteed or endorsed by the publisher.
References
1. Jia Y, Wang S, Wang S, Chen F. Femtosecond Laser Direct Writing of Flexibly Configured Waveguide Geometries in Optical Crystals: Fabrication and Application. Opto-Electronic Adv (2020) 3(10):190042. doi:10.29026/oea.2020.190042
2. Tervonen A, West BR, Honkanen S. Ion-exchanged Glass Waveguide Technology: A Review. Opt Eng (2011) 50(7):071107. doi:10.1117/1.3559213
3. Jia Y, Wang L, Chen F. Ion-cut Lithium Niobate on Insulator Technology: Recent Advances and Perspectives. Appl Phys Rev (2021) 8(1):011307. doi:10.1063/5.0037771
4. Zhang B, Tan D, Wang Z, Liu X, Xu B, Gu M, et al. Self-organized Phase-Transition Lithography for All-Inorganic Photonic Textures. Light Sci Appl (2021) 10:93. doi:10.1038/s41377-021-00534-5
5. Chen F, de Aldana JRV. Optical Waveguides in Crystalline Dielectric Materials Produced by Femtosecond-Laser Micromachining. Laser Photon Rev (2014) 8(2):251–75. doi:10.1002/lpor.201300025
6. Gross S, Dubov M, Withford MJ. On the Use of the Type I and II Scheme for Classifying Ultrafast Laser Direct-Write Photonics. Opt Express (2015) 23(6):7767–70. doi:10.1364/OE.23.007767
7. Lancaster DG, Gross S, Ebendorff-Heidepriem H, Kuan K, Monro TM, Ams M, et al. Fifty Percent Internal Slope Efficiency Femtosecond Direct-Written Tm^3+:ZBLAN Waveguide Laser. Opt Lett (2011) 36(9):1587–9. doi:10.1364/OL.36.001587
8. Burghoff J, Nolte S, Tünnermann A. Origins of Waveguiding in Femtosecond Laser-Structured LiNbO3. Appl Phys A (2007) 89(1):127–32. doi:10.1007/s00339-007-4152-0
9. Wu P, Jiang X, Zhang B, He S, Yang Q, Li X, et al. Mode-controllable Waveguide Fabricated by Laser-Induced Phase Transition in KTN. Opt Express (2020) 28(17):25633–41. doi:10.1364/OE.401407
10. Tan D, Sun X, Qiu J. Femtosecond Laser Writing Low-Loss Waveguides in Silica Glass: Highly Symmetrical Mode Field and Mechanism of Refractive index Change. Opt Mater Express (2021) 11(3):848–57. doi:10.1364/OME.419945
11. Rodenas A, Kar AK. High-contrast Step-index Waveguides in Borate Nonlinear Laser Crystals by 3D Laser Writing. Opt Express (2011) 19(18):17820–33. doi:10.1364/OE.19.017820
12. Ren Y, Chen F, Vázquez de Aldana JR. Near-infrared Lasers and Self-Frequency-Doubling in Nd:YCOB Cladding Waveguides. Opt Express (2013) 21(9):11562. doi:10.1364/oe.21.011562
13. Wu P, Zhu S, Hong M, Chen F, Liu H. Specklegram Temperature Sensor Based on Femtosecond Laser Inscribed Depressed Cladding Waveguides in Nd:YAG crystal. Opt Laser Technol (2019) 113:11–4. doi:10.1016/j.optlastec.2018.12.004
14. Lv J, Cheng Y, Yuan W, Hao X, Chen F. Three-dimensional Femtosecond Laser Fabrication of Waveguide Beam Splitters in LiNbO_3 crystal. Opt Mater Express (2015) 5(6):1274. doi:10.1364/OME.5.001274
15. Wang L, Zhang X, Li L, Lu Q, Romero C, Vázquez de Aldana JR, et al. Second Harmonic Generation of Femtosecond Laser Written Depressed Cladding Waveguides in Periodically Poled MgO:LiTaO3 crystal. Opt Express (2019) 27(3):2101–11. doi:10.1364/OE.27.002101
16. Jia Y, Vázquez de Aldana JR, Lu Q, Jaque D, Chen F. Second Harmonic Generation of Violet Light in Femtosecond-Laser-Inscribed BiB_3O_6 Cladding Waveguides. Opt Mater Express (2013) 3(9):1279–84. doi:10.1364/OME.3.001279
17. Burghoff J, Grebing C, Nolte S, Tünnermann A. Efficient Frequency Doubling in Femtosecond Laser-Written Waveguides in Lithium Niobate. Appl Phys Lett (2006) 89(8):081108. doi:10.1063/1.2338532
18. Okhrimchuk AG, Shestakov AV, Khrushchev I, Mitchell J. Depressed Cladding, Buried Waveguide Laser Formed in a YAG:Nd3+ crystal by Femtosecond Laser Writing. Opt Lett (2005) 30(17):2248–50. doi:10.1364/OL.30.002248
19. Liu H, Jia Y, Vázquez de Aldana JR, Jaque D, Chen F. Femtosecond Laser Inscribed Cladding Waveguides in Nd:YAG Ceramics: Fabrication, Fluorescence Imaging and Laser Performance. Opt Express (2012) 20(17):18620–9. doi:10.1364/OE.20.018620
20. Wu P, He S, Liu H. Annular Waveguide Lasers at 1064 Nm in Nd:YAG crystal Produced by Femtosecond Laser Inscription. Appl Opt (2018) 57:5420–4. doi:10.1364/AO.57.005420
21. Liao Y, Xu J, Cheng Y, Zhou Z, He F, Sun H, et al. Electro-optic Integration of Embedded Electrodes and Waveguides in LiNbO3 Using a Femtosecond Laser. Opt Lett (2008) 33(19):2281–3. doi:10.1364/OL.33.002281
22. Horn W, Kroesen S, Herrmann J, Imbrock J, Denz C. Electro-optical Tunable Waveguide Bragg Gratings in Lithium Niobate Induced by Femtosecond Laser Writing. Opt Express (2012) 20(24):26922–8. doi:10.1364/OE.20.026922
23. Zhang Q, Li M, Xu J, Lin Z, Yu H, Wang M, et al. Reconfigurable Directional Coupler in Lithium Niobate crystal Fabricated by Three-Dimensional Femtosecond Laser Focal Field Engineering. Photon Res (2019) 7(5):503. doi:10.1364/PRJ.7.000503
Keywords: optical waveguide, femtosecond laser direct writing, YAG crystal, beam splitter, integrated photonics device
Citation: Wu P, Yang S, Ren Y and Liu H (2021) Beam Splitters Fabricated by Nonlinear Focusing of Femtosecond Laser Writing in Pure YAG Crystal. Front. Phys. 9:719757. doi: 10.3389/fphy.2021.719757
Received: 03 June 2021; Accepted: 21 June 2021;
Published: 29 July 2021.
Edited by:
Jifeng Liu, Dartmouth College, United StatesReviewed by:
Rang Li, Helmholtz-Gemeinschaft Deutscher Forschungszentren (HZ), GermanyKaichen Xu, Zhejiang University, China
Copyright © 2021 Wu, Yang, Ren and Liu. This is an open-access article distributed under the terms of the Creative Commons Attribution License (CC BY). The use, distribution or reproduction in other forums is permitted, provided the original author(s) and the copyright owner(s) are credited and that the original publication in this journal is cited, in accordance with accepted academic practice. No use, distribution or reproduction is permitted which does not comply with these terms.
*Correspondence: Hongliang Liu, drliuhl@nankai.edu.cn