Innovation Through Heterochrony: An Amphioxus Perspective on Telencephalon Origin and Function
- Department of Biology, University of Victoria, Victoria, BC, Canada
Heterochrony has played a key role in the evolution of invertebrate larval types, producing “head larvae” in diverse taxa, where anterior structures are accelerated and specialized at the expense of more caudal ones. For chordates, judging from amphioxus, the pattern has been more one of repeated acceleration of adult features so that they function earlier in development, thus converting the ancestral larva, whether it was a head larva or not, into something progressively more chordate-like. Recent molecular data on gene expression patterns in the anterior nerve cord of amphioxus point to a similar process being involved in the origin of the telencephalon. As vertebrates evolved, a combination of acceleration and increasing egg size appears here to have allowed the development of a structure that would originally have emerged only gradually in the post-embryonic phase of the life history to be compressed into embryogenesis. The question then is what, in functional terms, makes the telencephalon so important to the survival of post-embryonic ancestral vertebrates that this was adaptively advantageous. A better understanding of the function this brain region performs in amphioxus may help provide the answer.
Introduction
One important way that development contributes to evolutionary innovation is through heterochrony, the process of accelerating or delaying some developmental events with respect to others (de Beer, 1971). This is most dramatic when it results in the emergence of a novel life history stage, as in the “head larvae” of various marine invertebrate taxa, where head structures are accelerated at the expense of more caudal ones, and become secondarily specialized to suit the larval mode of life. This accounts for features ranging from the suppressed trunk of many spiralians to the dipleurula-type larvae of echinoderms and hemichordates (Lacalli, 2005; Gonzalez et al., 2017), but the degree to which such changes are basal within bilaterians or are taxon-specific is a matter of debate (Strathmann, 2020). There also is a countertrend in larval specialization involving the acceleration of adult features so they function in the larva, a process known as adultation, which has occurred independently in multiple taxa (Jagersten, 1972).
How these ideas apply to chordates is not entirely clear, as chordate ancestry may never have included a true head larva like the dipleurula. But heterochrony would explain how locomotory specializations originally adapted for adult life (somites, nerve cord and notochord in the case of chordates) would progressively convert the ancestral larva, whatever it was like, into something anatomically more chordate-like. And, though newly hatched amphioxus have been justifiably referred to as “swimming heads” (Gilland and Baker, 1993), this is because development proceeds in an antero-posterior sequence, and only a limited number of anterior somites can be produced from the nutrient supply provided by the egg. With this framework in mind, the purpose of this brief account is to explore the implications of new data on another example of heterochrony involving what is evidently a chordate-specific neuroanatomical innovation, the telencephalon.
Telencephalon Origins and Ancestral Function
Most molecular data on amphioxus neural organization depend on studies on the early larva (reviewed by Holland and Holland, 2021). These reveal little about any putative telencephalon homolog because the antero-dorsal region from which it would necessarily form is largely undifferentiated at that time (Wicht and Lacalli, 2005). The question of whether amphioxus has such a homolog may now have been resolved by the observations of Benito-Gutierrez et al. (2021), who have identified markers associated with the telencephalon in a dorsal, pre-infundibular domain (termed the pars anterodorsalis, or PAD) that is insignificant in young larvae, but expands as the larvae mature, differentiating fully only in the adult. The molecular data reveal populations of glutamatergic and GABAergic neurons, both of which occur widely in the telencephalon, and of dopaminergic neurons, found in the vertebrate olfactory bulb and hypothalamus (Yamamoto and Vernier, 2011). There are similarities also with the telencephalon in the shape of the central canal and the pattern of thickening of the neural epithelium. The degree of homology is open to question, however, and the authors are suitably cautious in their interpretation. This is appropriate because the pre-infundibular neural domain, taken as a whole, maps to the apical plate of diverse invertebrate larvae (Tosches and Arendt, 2013; Marlow et al., 2014) and hence, predates chordates, which would account for the involvement of FoxG, a marker for apically-derived sensory derivatives such as photoreceptor organs (Toresson et al., 1998; Aldea et al., 2015; Liu and Satou, 2019). More specific to telencephalon are Emx and Nkx2.1, and Pax genes (Lichtneckert and Reichert, 2005; Molyneaux et al., 2007), but here too the expression could be as indicative of origin from a common domain as it is of structure-specific homology. A further problem is to determine whether the domain belongs to the dorsal part of the corresponding vertebrate prosomere or is an alar-plate hypothalamic derivative, which, based on current ideas about forebrain patterning (Puelles and Rubenstein, 2015; Yamamoto et al., 2017) is a possibility.
A crucial but missing piece of the puzzle is the function this rostral- and dorsal-most part of the brain performs in amphioxus. If it proved to share functions with the telencephalon, that would further support the case for homology, and provide some guidance regarding the ancestral function of this brain region in the last common ancestor of cephalochordates and vertebrates. Given the positioning of the olfactory tracts and their targets in vertebrates, in rostral forebrain (Kerman et al., 2013; Suryanarayana et al., 2021), the processing of olfactory inputs is very likely to have been a core function of this part of the ancestral brain. It is then suggestive that amphioxus has a late-developing pair of rostral nerve branches that project to (but also through) the PAD (Lacalli, 2002). Nothing is known of the sensory cells supplying these nerves beyond their location, at or near the tip of the rostrum (Poncelet and Shimeld, 2020), but this is precisely where amphioxus homologs of vertebrate olfactory neurons would be expected. And, the amphioxus genome does contain sequences for vertebrate-type olfactory receptors (Churcher and Taylor, 2009). Given the apparent underdevelopment of the PAD in all stages but mature adults, one could postulate a role, presumably olfactory, in the control of reproduction and spawning behavior. But there is as yet no evidence for this or any other olfactory involvement in the control of amphioxus behavior, a reminder of how neglected such aspects of amphioxus biology have been in recent decades.
The Life History Context
On the assumption Benito-Gutierrez et al. (2021) have indeed discovered an amphioxus homolog of the telencephalon, or at least a part of it, the life history context can be understood as shown in Figure 1: that in amphioxus, this brain region only begins its development once the pelagic feeding phase reaches sufficient size, and may only function in the mature adult. For vertebrates, in contrast, a combination of increased yolk supply and developmental acceleration has compressed the developmental sequence so it can be completed within the period of embryogenesis. This implies that having a functioning telencephalon at or shortly after hatching was of such benefit to basal vertebrates that the diversion of nutrient required to achieve this was justified by increased hatchling survival. The eyes fit this same pattern, as they are disproportionately large in the young stages of many vertebrates, including, for example, many fish larvae. The advantage of precocious development to full size is understandable in the case of the eye, as a large optic cup fitted with a lens has clear advantages in terms of resolution and image-formation over a small cluster of photoreceptors, however, shaped and positioned. In consequence, if the ancestral condition was one where a simple photoreceptor array developed progressively as the animal grew, adding elements and reshaping itself as size permitted, there should be strong selective pressure to shift the point where an image of optimal resolution could be produced to the earliest possible stage in the life history.
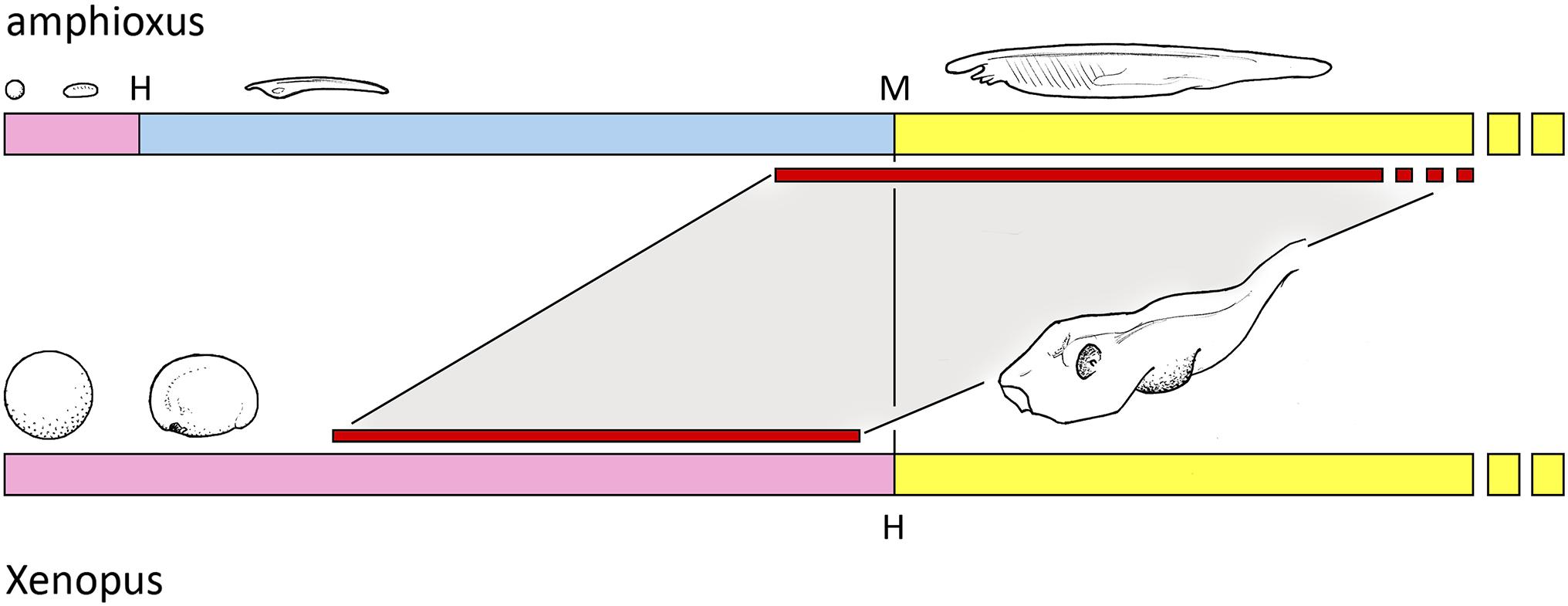
Figure 1. The early life history of amphioxus compared with a representative aquatic vertebrate, Xenopus in this case. The embryonic stage (in pink) produces a hatching larva (at H), followed by a pelagic feeding phase (blue) that metamorphoses (M) to a juvenile that is essentially a small adult with adult habits (yellow). Embryogenesis in Xenopus also produces a larva, but is here shown in yellow because it corresponds less with the amphioxus larva than the juvenile in size and anatomical complexity, and the term larva is problematic anyway if, as here, the stages in question likely have independent evolutionary origins. Two trends are evident. First, that vertebrate eggs and embryos are orders of magnitude larger in volume, and so can be far more fully developed at hatching, and second, that this allows features that can only develop progressively in amphioxus to be incorporated into embryogenesis in vertebrates. The focus in this account is the telencephalon and its putative amphioxus homolog, whose periods of development, inferred for amphioxus from data reported by Benito-Gutierrez et al. (2021), are shown by the red bars.
The same argument could apply to processing olfactory input. Olfaction is crucial for the detection of predators and kin in fish larvae of various types, a function that involves olfactory imprinting (Chivers and Smith, 1994; Gerlach and Wullimann, 2021). Whether this is considered a rudimentary form of learning or more akin to memory, it implies integrative functions of some complexity requiring a dedicated circuitry and a more than minimal tissue mass. Hence, like the eye, there would be some justification for supposing that precocious differentiation, rather than a gradual increase in size and cell numbers, could have been the better option from an adaptive standpoint. Conversely, for early vertebrates, a fully functional telencephalon may have been needed, even in young hatchlings, to meet the demands of an emerging predatory mode of life, e.g., to coordinate the visual tracking and capture of prey. Amphioxus would then be important primarily as a benchmark, defining a starting point for these and other subsequent changes.
Neuronal Cell Types and Their Origins
It is worth considering also the evolutionary origin of the cell types identified by Benito-Gutierrez et al. (2021), especially the glutamatergic and GABAergic neurons which by implication, would be related by homology to the main excitatory and inhibitory cell classes, respectively, in vertebrate cortex. Two categories of dorsal post-infundibular amphioxus neurons, the translumenal and dorsal bipolar neurons, are noteworthy here for being apico-basally inverted (Lacalli and Kelly, 2003), a feature also characteristic of cortical neurons (Barnes and Polleux, 2009). However, both types of amphioxus neurons appear to be absent from the pre-infundibular region of the adult nerve cord (Castro et al., 2015), which may rule out a direct relation, through homology, with particular classes of telencephalic neurons. It would be useful to know whether the glutamatergic neurons identified by Benito-Gutierrez et al. (2021) will, on further investigation, show signs of inversion, or whether this feature is, for the telencephalon at least, a vertebrate innovation. In either case, and despite similar transmitters being involved, it is entirely possible that the dorsal neurons populating the cerebral cortex are only distantly related in evolutionary terms to those in midbrain and hindbrain, i.e., tectum and cerebellum, and possess unique functional capabilities that account for the division of labor between these structures. Molecular criteria for distinguishing among the relevant cell types are only beginning to be applied across vertebrates (e.g., Tosches and Laurant, 2019), but in future could be usefully extended to include amphioxus.
Discussion
The results of Benito-Gutierrez et al. (2021) can be interpreted as indicting strongly that the telencephalon, as a structure with particular functions, is a comparatively late innovation that is chordate-specific in its elaboration. Understanding the sequence of events that led to its emergence as a major brain region in vertebrates then depends on knowing its function in basal chordates. A role in the processing of olfactory input probably predates other functions, but there could be additional tasks preformed by this brain region, also traceable back to the common ancestor of amphioxus and vertebrates, of which we are as yet unaware. To resolve this issue, more research on adult amphioxus will be required, both on behavior and sensory physiology, but especially on neuroanatomy at the cellular level, directed toward compiling an inventory of the neuronal cell types in the rostral brain, their morphology and synaptic connections. Ideally one would like to have a complete map of synaptic patterns, i.e., the connectome, for this brain region. This has already been done in large part for young larvae, and adult stages would not present much more of a technical challenge given recent improvements in methodology (Landhuis, 2020). Compared to the brain of a mouse or larval zebrafish, an analysis of the amphioxus brain is orders of magnitude less demanding, with the promise of meaningful results in a correspondingly shorter time.
Data Availability Statement
There is no data beyond that included in the article; further inquiries can be directed to the corresponding author.
Author Contributions
The author confirms he is the sole contributor to this work and has approved it for publication.
Funding
Funds to support this work were received from the L. G. Harrison Research Trust.
Conflict of Interest
The author declares that the research was conducted in the absence of any commercial or financial relationships that could be construed as a potential conflict of interest.
Acknowledgments
I thank Nick and Linda Holland for their comments, Detlev Arendt for providing information on the amphioxus PAD before publication, and Riley Lacalli for assisting with the figure. I take this opportunity also to acknowledge R. J. F. (Jan) Smith, who introduced me to the alarming world of fish behavior. His untimely death cut short plans for a joint, collaborative investigation of amphioxus olfaction.
References
Aldea, D., Leon, A., Bertrand, S., and Escriva, H. (2015). Expression of Fox genes in the cephalochordate Branchiostoma lanceolatum. Front. Ecol. Evol. 3:80. doi: 10.3389/fevo.2015.00080
Barnes, A. P., and Polleux, F. (2009). Establishment of axon-dendrite polarity in developing neurons. Annu. Rev. Neurosci. 32, 347–381. doi: 10.1146/annurev.neuro.31/060407.125536
Benito-Gutierrez, E., Gattoni, G., Stemmer, M., Rohr, S. D., Schuhmacher, L. N., Tang, J., et al. (2021). The dorsoanterior brain of adult amphioxus shares similarities in expression profile and neuronal composition with the vertebrate telencephalon. BMC Biol. 19:110. doi: 10.1186/s12915-021-01045-w
Castro, A., Becerra, M., Manso, M. J., and Anadon, R. (2015). Neuronal organization in the brain in the adult amphioxus (Branchiostoma lanceolatum): a study with acetylated tubulin immunohistochemistry. J. Comp. Neurol. 523, 2211–2232. doi: 10.1002/cne.23785
Chivers, D. P., and Smith, R. J. F. (1994). The role of experience and chemical signaling in predator recognition by fathead minnows. J. Fish. Biol. 44, 273–285. doi: 10.1111/j.1095-8649.1994.tb01205.x
Churcher, A. M., and Taylor, J. S. (2009). Amphioxus (Branchiostoma floridae) has orthologs of vertebrate odorant receptors. BMC Evol. Biol. 9:242. doi: 10.1186/1471-2148-9-242
Gerlach, G., and Wullimann, M. F. (2021). Neural pathways of olfactory kin imprinting and kin recognition in zebrafish. Cell Tiss. Res. 383, 273–287. doi: 10.1007/s00441-020-03378-4
Gilland, E., and Baker, R. (1993). Conservation of neuroepithelial and mesodermal segments in the embryonic vertebrate head. Acta Anat. 148, 110–123. doi: 10.1159/000147530
Gonzalez, P., Uhlinger, K. R., and Lowe, C. J. (2017). The adult body plan of indirect developing hemichordates develops by adding a Hox-patterned trunk to an anterior larval territory. Curr. Biol. 27, 87–95. doi: 10.1016/j.cub.2016.10.047
Holland, L. Z., and Holland, N. D. (2021). Cephalochordates: a window into vertebrate origins. Curr. Top. Dev. Biol. 141, 119–147. doi: 10.1016/bs.ctdb.2020.07.001
Jagersten, G. (1972). Evolution of the Metazoan Life Cycle: A Comprehensive Theory. New York, NY: Academic Press.
Kerman, F., Franco, L. M., Wyatt, L., and Yaksi, E. (2013). Neural circuits mediating olfactory-driven behavior in fish. Front. Neural Circuits 7:62. doi: 10.3389/fncr.2013.00062
Lacalli, T. C. (2002). Sensory pathways in amphioxus larvae I. constituent fibres of the rostral and anterodorsal nerves, their targets and evolutionary significance. Acta Zool. 83, 149–166. doi: 10.1046/j.1463-6395.2003.00109.x
Lacalli, T. C. (2005). Protochordate body plan and the evolutionary role of larvae: old controversies resolved? Can. J. Zool. 83, 216–224. doi: 10.1139/Z04-162
Lacalli, T. C., and Kelly, S. J. (2003). Sensory pathways in amphioxus larvae II. dorsal tracts and translumenal cells. Acta Zool. 84, 1–13. doi: 10.1046/j.1463-6395.2002.00065.x
Landhuis, E. (2020). Probing fine-scale connections in the brain. Nature 586, 631–633. doi: 10.1038/d41586-020-02947-5
Lichtneckert, R., and Reichert, H. (2005). Insights into the urbilaterian brain: conserved genetic patterning mechanisms in insect and vertebrate brain development. Heredity 94, 465–477. doi: 10.1038/sj.hdy.6800664
Liu, B., and Satou, Y. (2019). Foxg specifies sensory neurons on the anterior neural plate border of the ascidian embryo. Nat. Comm. 10:4911. doi: 10.1038/s41467-019-12839-6
Marlow, H., Tosches, M. A., Tomer, R., Steinmetz, P. R., Lauri, A., and Arendt, D. (2014). Larval body patterning and apical organs are conserved in animal evolution. BMC Biol. 12:7. doi: 10.1186/1741.7007.12.7
Molyneaux, B. J., Arlotta, P., Menezes, J. R. L., and Macklis, J. D. (2007). Neuronal subtype specification in the cerebral cortex. Nat. Rev. Neurosci. 8, 427–437. doi: 10.1038/nrn2151
Poncelet, G., and Shimeld, S. M. (2020). The evolutionary origin of the vertebrate olfactory system. Open Biol. 10:200330. doi: 10.1098/rsob.200330
Puelles, L., and Rubenstein, J. L. R. (2015). A new scenario of hypothalamic organization: rationale of new hypotheses introduced in the updated prosomeric model. Front. Neuroanat. 9:27. doi: 10.3389/fnana.2015.00027
Strathmann, R. R. (2020). Multiple origins of feeding head larvae by the early Cambrian. Can. J. Zool. 98, 761–776. doi: 10.1139/cjz-2019-0284
Suryanarayana, S. M., Perez-Fernandez, J., Robertson, B., and Grillner, S. (2021). Olfaction in lamprey revisited – dual projections of mitral and tufted cells. Cell Rep. 34:108596. doi: 10.1016/j.celrep.2020.108596
Toresson, H., Matinez-Barbera, J. P., Bardsley, A., Caubit, X., and Krauss, S. (1998). Conservation of BF-1 expression in amphioxus and zebrafish suggests evolutionary ancestry of anterior cell types that contribute to vertebrate telencephalon. Dev. Genes. Evol. 208, 431–439. doi: 10.1007/s004270050200
Tosches, M. A., and Arendt, D. (2013). The bilaterian forebrain: an evolutionary chimera. Curr. Opin. Neurobiol. 23, 1–10. doi: 10.1016/j.conb.2013.09.005
Tosches, M. A., and Laurant, G. (2019). Evolution of neural identity in cerebral cortex. Curr. Opin. Neurobiol. 56, 199–208. doi: 10.1016/j.conb.2019.04.009
Wicht, H., and Lacalli, T. C. (2005). The nervous system of amphioxus: structure, development, and evolutionary signficance. Can. J. Zool. 83, 122–150. doi: 10.1139/Z04-163
Yamamoto, K., and Vernier, P. (2011). The evolution of dopamine systems in chordates. Front. Neuroanat. 5:21. doi: 10.3389/fnana.2011.00021
Keywords: developmental timing, head larvae, forebrain prosomeres, olfaction, cortical neurons
Citation: Lacalli T (2021) Innovation Through Heterochrony: An Amphioxus Perspective on Telencephalon Origin and Function. Front. Ecol. Evol. 9:666722. doi: 10.3389/fevo.2021.666722
Received: 16 February 2021; Accepted: 29 April 2021;
Published: 04 June 2021.
Edited by:
Juan Pascual-Anaya, Malaga University of Málaga, SpainReviewed by:
Hector Escriva, Centre National de la Recherche Scientifique (CNRS), FranceSebastian Shimeld, University of Oxford, United Kingdom
Copyright © 2021 Lacalli. This is an open-access article distributed under the terms of the Creative Commons Attribution License (CC BY). The use, distribution or reproduction in other forums is permitted, provided the original author(s) and the copyright owner(s) are credited and that the original publication in this journal is cited, in accordance with accepted academic practice. No use, distribution or reproduction is permitted which does not comply with these terms.
*Correspondence: Thurston Lacalli, lacalli@uvic.ca