Abstract
In this paper, an analytical matrix method is presented to drive closed-form characteristic equations for natural frequencies of finite monoatomic and diatomic metamaterials with various boundary conditions. Here, we extend the matrix method introduced by Louck for monoatomic lattice chains. The proposed method is used to calculate the vibration frequencies of the monoatomic metamaterials with fixed–fixed, fixed-free and free-free boundary conditions. In addition, the natural frequencies of fixed–fixed diatomic metamaterials are calculated. The existence of band gaps in the frequencies of the metamaterials is numerically shown.







Similar content being viewed by others
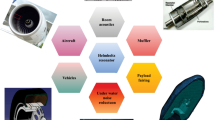
References
Yao S, Zhou X, Hu G (2008) Experimental study on negative effective mass in a 1D mass–spring system. New J Phys 10:043020
Huang HH, Sun CT, Huang GL (2009) On the negative effective mass density in acoustic metamaterials. Int J Eng Sci 47:610–617
Cselyuszka N, Sečujski M, Crnojević-Bengin V (2015) Novel negative mass density resonant metamaterial unit cell. Phys Lett A 379:33–36
Henyš P, Vomáčko V, Ackermann M, Sobotka J, Solfronk P, Šafka J, Čapek L (2019) Normal and shear behaviours of the auxetic metamaterials: homogenisation and experimental approaches. Meccanica 54:831–839
Shaat M, Wagih A (2020) Hinged-3D metamaterials with giant and strain-independent Poisson’s ratios. Sci Rep 10:2228
Wang X (2014) Dynamic behaviour of a metamaterial system with negative mass and modulus. Int J Solids Struct 51:1534–1541
Sridhar A, Liu L, Kouznetsova VG, Geers MGD (2018) Homogenized enriched continuum analysis of acoustic metamaterials with negative stiffness and double negative effects. J Mech Phys Solids 119:104–117
Li QQ, He ZC, Li E (2019) Dissipative multi-resonator acoustic metamaterials for impact force mitigation and collision energy absorption. Acta Mech 230:2905–2935
Xu X, Barnhart MV, Fang X, Wen J, Chen Y, Huang G (2019) A nonlinear dissipative elastic metamaterial for broadband wave mitigation. Int J Mech Sci 164:105159
Hu G, Tang L, Das R, Gao S, Liu H (2017) Acoustic metamaterials with coupled local resonators for broadband vibration suppression. AIP Adv 7:025211
Basta EE, Ghommem M, Emam SA (2020) Vibration suppression and optimization of conserved-mass metamaterial beam. Int J Nonlinear Mech 120:103360
Wagner PR, Dertimanis VK, Antoniadis IA, Chatzi EN (2016) On the feasibility of structural metamaterials for seismic-induced vibration mitigation. Int J Earthq Impact Eng 1:20–56
Yang X, Yin J, Yu G, Peng L, Wang N (2015) Acoustic superlens using Helmholtz-resonator-based metamaterials. Appl Phys Lett 107:193505
Chen M, Jiang H, Zhang H, Li D, Wang Y (2018) Design of an acoustic superlens using single-phase metamaterials with a star-shaped lattice structure. Sci Rep 8:1861
Szajek K, Sumelka W (2019) Discrete mass-spring structure identification in nonlocal continuum space-fractional model. Eur Phys J Plus 134:448
Failla G, Ghavanloo E (2021) Nonlocal approaches to the dynamics of metamaterials. In: Ghavanloo et al (eds) Size-Dependent continuum mechanics approaches. Springer, Berlin
Huang HH, Sun CT (2012) Anomalous wave propagation in a one-dimensional acoustic metamaterial having simultaneously negative mass density and Young’s modulus. J Acoust Soc Am 132:2887–2895
Li B, Tan KT (2016) Asymmetric wave transmission in a diatomic acoustic/elastic metamaterial. J Appl Phys 120:075103
Bacigalupo A, Gambarotta L (2017) Wave propagation in non-centrosymmetric beam-lattices with lumped masses: discrete and micropolar modeling. Int J Solids Struct 118:128–145
Bacigalupo A, Lepidi M (2018) Acoustic wave polarization and energy flow in periodic beam lattice materials. Int J Solids Struct 147:183–203
Lepidi M, Bacigalupo A (2019) Wave propagation properties of one-dimensional acoustic metamaterials with nonlinear diatomic microstructure. Nonlinear Dyn 98:2711–2735
Jaberzadeh M, Li B, Tan KT (2019) Wave propagation in an elastic metamaterial with anisotropic effective mass density. Wave Motion 89:131–141
Wang YF, Liang JW, Chen AL, Wang YS, Laude V (2019) Wave propagation in one-dimensional fluid-saturated porous metamaterials. Phys Rev B 99:134304
Ghavanloo E, Fazelzadeh SA (2019) Wave propagation in one-dimensional infinite acoustic metamaterials with long-range interactions. Acta Mech 230:4453–4461
Zhu R, Huang GL, Hu GK (2012) Effective dynamic properties and multi-resonant design of acoustic metamaterials. J Vib Acoust 34:031006
Sugino C, Leadenham S, Ruzzene M, Erturk A (2016) On the mechanism of bandgap formation in locally resonant finite elastic metamaterials. J Appl Phys 120:134501
Al Ba’ba’a H, Nouh M, Singh T (2017) Formation of local resonance band gaps in finite acoustic metamaterials: a closed-form transfer function model. J Sound Vib 410:429–446
Shen XH, Sun CT, Barnhart MV, Huang GL (2018) Analysis of dynamic behavior of the finite elastic metamaterial-based structure with frequency-dependent properties. J Vib Acoust 140:031012
Al Ba’ba’a H, DePauw D, Singh T, Nouh M (2018) Dispersion transitions and pole-zero characteristics of finite inertially amplified acoustic metamaterials. J Appl Phys 123:105106
Mu D, Shu H, An S, Zhao L (2020) Free and steady forced vibration characteristics of elastic metamaterial beam. AIP Adv 10(03530):4
Failla G, Santoro R, Burlon A, Russillo AF (2020) An exact approach to the dynamics of locally-resonant beams. Mech Res Commun 103:103460
El-Borgi S, Fernandes R, Rajendran P, Yazbeck R, Boyd JG, Lagoudas DC (2020) Multiple bandgap formation in a locally resonant linear metamaterial beam: theory and experiments. J Sound Vib 488:115647
Louck JD (1962) Exact normal modes of oscillation of a linear chain of identical particles. Am J Phys 30:585–590
Williams KS (1991) The nth power of a 2×2 matrix. Math Mag 65:336–336
Acknowledgements
This study is dedicated to Prof. Elias C. Aifantis on occasion of his 70th birthday.
Author information
Authors and Affiliations
Corresponding author
Ethics declarations
Conflict of interest
The authors declare that there are no conflicts of interest.
Additional information
Publisher's Note
Springer Nature remains neutral with regard to jurisdictional claims in published maps and institutional affiliations.
Rights and permissions
About this article
Cite this article
Ghavanloo, E., Fazelzadeh, S.A. An analytical approach for calculating natural frequencies of finite one-dimensional acoustic metamaterials. Meccanica 56, 1819–1829 (2021). https://doi.org/10.1007/s11012-021-01332-4
Received:
Accepted:
Published:
Issue Date:
DOI: https://doi.org/10.1007/s11012-021-01332-4