Abstract
One-dimensional, unsteady gaseous detonation propagation in a non-homogeneous medium is investigated numerically using the reactive, compressible Navier–Stokes equations with detailed chemistry. The effect of concentration inhomogeneity on the pulsating mode is modeled by a sinusoidal distribution of H2 mole fraction in a H2–O2 mixture. The mixture inhomogeneity, varied by changing the disturbance frequency and amplitude, has significant effects on the pulsating behavior of the detonation due to the interaction of the leading shock with the local gradient. Initially exhibiting a four-period pulsation, the detonation wave entering a non-homogeneous medium can adapt and re-establish into a new propagation mode. For a fixed, large-amplitude sinusoidal disturbance, the period-doubling limit cycle is prone to be destroyed, resulting in a chaotic mode for the propagating detonation front in the non-homogeneous mixture; lowering the disturbance frequency also favors a transition from a periodic pulsation to a chaotic one. When the disturbance amplitude decreases, the propagating detonation can transit quickly to a new pulsating behavior, which tends to be more regular. For a very small amplitude of inhomogeneous variation, it is found that the frequency corresponding to the wavelength close to that of the intrinsic pulsation in the uniform mixture makes the original four-period mode become a double-period mode; for a frequency less than this value, the double-period mode is prone to become more unstable. Consequently, this demonstrates that inhomogeneity could have a positive effect on stabilizing a pulsating detonation.












Similar content being viewed by others
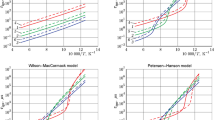
References
Lee, J.H.S.: The Detonation Phenomenon. Cambridge University Press, Cambridge (2008) https://doi.org/10.1017/CBO9780511754708
Clavin, P., Searby, G.: Combustion Waves and Fronts in Flow. Cambridge University Press, Cambridge (2016). https://doi.org/10.1017/CBO9781316162453.003
McVey, J.B., Toong, T.Y.: Mechanism of instabilities of exothermic hypersonic blunt body flows. Combust. Sci. Tech. 3, 63–76 (1971). https://doi.org/10.1080/00102207108952273
Clavin, P., Williams, F.A.: Dynamics of planar gaseous detonations near Chapman–Jouguet conditions for small heat release. Combust. Theor. Model. 6, 127–139 (2002). https://doi.org/10.1088/1364-7830/6/1/307
Erpenbeck, J.J.: Stability of steady-state equilibrium detonations. Phys. Fluids 5, 604–614 (1962). https://doi.org/10.1063/1.1706664
Sharpe, G.J., Falle, S.A.E.G.: One-dimensional numerical simulations of idealized detonations. Proc. R. Soc. Lond. Ser. A Math. Phys. Sci. 455, 1203–1214 (1999). https://doi.org/10.1098/rspa.1999.0355
Short, M., Sharpe, G.J.: Pulsating instability of detonations with a two-step chain-branching reaction model: Theory and numerics. Combust. Theor. Model. 7, 401–416 (2003). https://doi.org/10.1088/1364-7830/7/2/311
Radulescu, M.I., Ng, H.D., Lee, J.H.S., Varatharajan, B.: The effect of argon dilution on the stability of acetylene-oxygen detonations. Proc. Combust. Inst. 29, 2825–2831 (2002). https://doi.org/10.1016/S1540-7489(02)80345-5
Yungster, S., Radhakrishan, K.: Pulsating one-dimensional detonations in hydrogen–air mixtures. Combust. Theor. Model. 8, 745–770 (2004). https://doi.org/10.1088/1364-7830/8/4/005
Thomas, G.O., Sutton, P., Edwards, D.H.: The behavior of detonation waves at concentration gradients. Combust. Flame. 84, 312–322 (1991). https://doi.org/10.1016/0010-2180(91)90008-Y
Kuznetsov, M.S., Alekseev, V.I., Dorofeev, S.B., Matsukov, I.D., Boccio, J.L.: Detonation propagation, decay, and reinitiation in nonuniform gaseous mixtures. Symposium (International) on Combustion 27(2), 2241–2247 (1998). https://doi.org/10.1016/S0082-0784(98)80073-8
Ishii, K., Kojima, M.: Behavior of detonation propagation in mixtures with concentration gradients. Shock Waves 17(1–2), 95–102 (2007). https://doi.org/10.1007/s00193-007-0093-y
Boeck, L.R., Berger, F.M., Hasslberger, J., Sattelmayer, T.: Detonation propagation in hydrogen–air mixtures with transverse concentration gradients. Shock Waves 26(2), 181–192 (2016). https://doi.org/10.1007/s00193-015-0598-8
Calhoon, W., Sinha, N.: Detonation wave propagation in concentration gradients. 43th Aerospace Sciences Meeting and Exhibit, Reno, NV, AIAA Paper 2005-1167 (2005). https://doi.org/10.2514/6.2005-1167
Kessler, D., Gamezo, V., Oran, E.: Wave structures and irregular detonation cells in methane-air mixtures with concentration gradients. 49th Aerospace Sciences Meeting including the New Horizons Forum and Aerospace Exposition, Orlando, FL, AIAA Paper 2011-798 (2011). https://doi.org/10.2514/6.2011-798
Ettner, F., Vollmer, K.G., Sattelmayer, T.: Mach reflection in detonations propagating through a gas with a concentration gradient. Shock Waves 23(3), 201–206 (2013). https://doi.org/10.1007/s00193-012-0385-8
Oran, E.S., Jones, D.A., Sichel, M.: Numerical simulations of detonation transmission. Proc. R. Soc. Lond. Ser. A Math. Phys. Sci. 436, 267–297 (1992). https://doi.org/10.1098/rspa.1992.0018
Mi, X.C., Timofeev, E.V., Higgins, A.J.: Effect of spatial discretization of energy on detonation wave propagation. J. Fluid Mech. 817, 306–338 (2016). https://doi.org/10.1017/jfm.2017.81
Han, W.H., Wang, C., Law, C.K.: Pulsation in one-dimensional H2–O2 detonation with detailed reaction mechanism. Combust. Flame 200, 242–261 (2019). https://doi.org/10.1016/j.combustflame.2018.11.024
Wang, C., Shu, C.W., Han, W.H., Ning, J.G.: High resolution WENO simulation of 3D detonation waves. Combust. Flame 160, 447–462 (2013). https://doi.org/10.1016/j.combustflame.2012.10.002
He, L.T., Lee, J.H.S.: The dynamical limit of one-dimensional detonations. Phys. Fluids 7, 1151–1158 (1995). https://doi.org/10.1063/1.868556
Austin, J.M., Pintgen, F., Shepherd, J.E.: Reaction zones in highly unstable detonations. Proc. Combust. Inst. 30, 1849–1857 (2005). https://doi.org/10.1016/j.proci.2004.08.157
Kee, R.J., Rupley, F.M., Miller, J.A.: Chemkin II: A Fortran chemical kinetics package for the analysis of gas phase chemical kinetics. Technical Report, SAND89-8009B. Sandia National Laboratories (1992). https://doi.org/10.2172/481621
Kee, R.J., Dixon-Lewis, G., Warnatz, J., Coltrin, M.E., Miller, J.A., Moffat H.K.: A Fortran computer code package for the evaluation of gas-phase multicomponent transport properties. Sandia National Laboratories Report SAND86-8246. 13, 80401–1887 (1986)
Sánchez, A.L., Williams, F.A.: Recent advances in understanding of flammability characteristics of hydrogen. Prog. Energ. Combust. 41, 1–55 (2014). https://doi.org/10.1016/j.pecs.2013.10.002
Han, W.H., Ma, W.J., Qian, C.G., Wen, J., Wang, C.: Bifurcation of pulsation instability in one-dimensional H2–O2 detonation with detailed reaction mechanism. Phys. Rev. Fluids 4(10), 103202 (2019). https://doi.org/10.1103/physrevfluids.4.103202
Acknowledgements
The research was sponsored by the National Natural Science Foundation of China under Grants 11972090,11732003, and U1830139, the Science and Technology on Transient Impact Laboratory Foundation (Grant No. 6142606182104), and the State Key Laboratory of Explosion Science and Technology. WH was in addition supported by a European Commission for the Marie Curie International Fellowships Grant “TurbDDT (Grant No. 793072).”
Author information
Authors and Affiliations
Corresponding authors
Additional information
Communicated by G. Ciccarelli.
Publisher's Note
Springer Nature remains neutral with regard to jurisdictional claims in published maps and institutional affiliations.
This paper is based on work that was presented at the 25th International Colloquium on the Dynamics of Explosions and Reactive Systems, Beijing, China, July 28–August 2, 2019.
Rights and permissions
About this article
Cite this article
Ma, W.J., Wang, C. & Han, W.H. Effect of concentration inhomogeneity on the pulsating instability of hydrogen–oxygen detonations. Shock Waves 30, 703–711 (2020). https://doi.org/10.1007/s00193-020-00976-7
Received:
Revised:
Accepted:
Published:
Issue Date:
DOI: https://doi.org/10.1007/s00193-020-00976-7