Abstract
Hydrogen transition wavelengths computed with the familiar Balmer–Bohr formula are different than those reported in standard reference sources. The origin of this discrepancy, which is likely not well-known to instructors and students, is explained.
Export citation and abstract BibTeX RIS
Every student of physics and chemistry, and many of general-level astronomy, will encounter the Bohr model for the hydrogen atom during their classes. One of the most famous results of this theory is an expression for the wavelength of light emitted when an electron transits from one permitted orbit to another. If the initial orbit is ninitial and the final one nfinal (ninitial > nfinal ), the emission wavelength is given by the formula
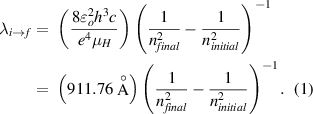
The symbols in the prefactor have their usual meanings: o
is the permittivity of free space, h is Planck's constant, c is the speed of light, e is the electronic charge, and μH
is the so-called reduced mass of hydrogen,

where mp and me are respectively the masses of the proton and electron. The reduced mass accounts for the motion of the proton and electron around their mutual centre of mass. The inverse of the prefactor is the Rydberg constant for hydrogen.
Equation (1) is now known as the Balmer–Bohr formula, a generalization of an empirical expression for the visible-wavelength spectral lines of hydrogen found by Johann Balmer in 1885. The visible-wavelength lines, what we now call the Balmer lines, correspond to transitions down to nfinal = 2 from higher-n orbits. Precise modern values for the physical constants and the resulting reduced mass and Rydberg constant are listed in table 1.
Table 1. Physical constants.
Name | Symbol | Value | Units |
---|---|---|---|
Speed of light | c | 2.997 924 58 × 108 | m s−1 |
Planck's constant | h | 6.626 070 15 × 10–34 | J s |
Electron charge | e | 1.602 176 634 × 10–19 | C |
Permittivity constant |
![]() | 8.854 187 812 8 × 10–12 | F m−1 |
Electron mass | me | 9.109 383 701 5 × 10–31 | kg |
Proton mass | mp | 1.672 621 923 7 × 10–27 | kg |
Neutron mass | mn | 1.674 927 498 × 10–27 | kg |
Rydberg constant | RH | 10 967 758.3 | m−1 |
Reduced mass | μH | 9.104 425 278 × 10–31 | kg |
Source: National Institute of Standards and Technology Recommended Values of the Fundamental Physical Constants: 2018. https://physics.nist.gov/cuu/pdf/wall_2018.pdf
While recently programming a spreadsheet to compute transition wavelengths for class use, I was dismayed to find my results for the Balmer series coming in 1–2 Å greater than standard reference values; see table 2. At first I thought I had entered one or more of the physical constants incorrectly, but this proved not to be so. The number of significant figures used for the constants was such that the problem could not have been round-off error.
Table 2. Reference, computed, and refraction-corrected Balmer wavelengths.
ninitial | Text value a (Å) | Equation (1) (Å) | Equation (1) corrected (Å) |
---|---|---|---|
3 | 6562.80 | 6564.70 | 6562.90 |
4 | 4861.32 | 4862.74 | 4861.40 |
5 | 4340.46 | 4341.73 | 4340.54 |
6 | 4101.73 | 4102.94 | 4101.81 |
Infinity | 3646 | 3647.05 | 3646.05 |
aCox A N 2000 Allen's Astrophysical Quantities 4th edn (New York: Springer) p 70.
A little searching soon revealed the culprit: published and online wavelength tabulations typically quote optical region wavelengths for what would be measured in air, while those given by equation (1) would be for a vacuum. The index of refraction of air, nair ∼ 1.0003, is only marginally greater than that of a vacuum, but sufficient to cause the difference. What I needed to do was to convert my vacuum wavelengths to air wavelengths via λair = λvac /nair , but such things are never as simple as they seem: the index of refraction of air is a function of pressure, humidity, carbon dioxide content, and wavelength itself. Fortunately, the United States National Institute of Standards and Technology maintains an online app which calculates the index of refraction for a given combination of vacuum wavelength and atmospheric conditions [1].
For the range of wavelengths of interest here, this app gives nair ∼ 1.00027 to 1.00028 for a temperature of 20 °C, standard pressure (101.325 kPa), 50% relative humidity, and CO2 content 450 ppm. Adopting 1.000275 as an effective average value, the last column in table 2 lists the corresponding air wavelengths, which agree with their textbook counterparts within 0.1 Å. There will always be minor discrepancies with the Balmer–Bohr formula because it is non-relativistic, and spectral lines actually have fine structure; there are no single wavelengths for these lines.
This issue will be well-known to spectroscopists and atomic physicists, but probably not to non-specialist instructors and ambitious students who want to check the numbers for themselves. Even with a long-familiar result, there is always something new to learn.
Biographies
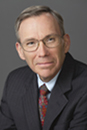
B Cameron Reed is an emeritus professor of physics at Alma College. He completed his Ph.D. in physics at the University of Waterloo in Canada in 1984. He joined the faculty of Alma College in 1992. He is an expert in nuclear weapons, the Manhattan project, and quantum physics.