Performance Enhancement of Permeable Asphalt Mixtures With Recycled Aggregate for Concrete Pavement Application
- 1School of Civil Engineering and Architecture, Nanchang University, Jiangxi, China
- 2School of Civil and Environmental Engineering, University of Technology Sydney, Sydney, NSW, Australia
- 3School of Built Environment, Western Sydney University, Sydney, NSW, Australia
- 4Department of Civil, Construction and Environmental Engineering, Iowa State University, Ames, IA, United States
The incorporation of recycled concrete aggregate (RCA) in permeable asphalt mixtures (PAMs) is an efficient method of utilizing construction demolished waste. It not only conforms to the trend of building sponge cities, but also alleviates the problem of overexploitation of natural aggregate resources. As the performance of PAM containing recycled aggregate is not comparable to natural aggregate, modification treatments and the addition of hybrid fibers are adopted as two enhancement methods to improve the performance of PAM with RAC in this study. It is found that replacing natural aggregate with recycled aggregate increases the optimum asphalt content (OAC) but decreases the residual stability. The OAC is increased by 45% when the RCA ratio is 100%, whereas applying silicone resin can give a 16.2% decrease in the OAC. Enhancing RCA with silicone resin can increase the water stability to be comparable with natural aggregate. Moreover, with modification treatment using calcium hydroxide solution, the mechanical strength of PAM is enhanced to even higher than that of natural coarse aggregate mixture alone. Improvements in both mechanical strength and water stability are also achieved by strengthening recycled aggregate with cement slurry, although the performance is less effective than using silicone resin. With the increase in the content of RCA, the permeability coefficients of PAM first decrease and then exhibit an increasing trend. The results indicate that the PAM with RCA and modification treatments can perform satisfactorily as a pavement material in practice. Applying probable modification, PAM incorporating RCA meets the criteria for use in concrete pavement applications.
Introduction
With the rapid development of the global economy, colossal amounts of waste have been generated by burgeoning populations, expanding industries, and tremendous urban growth. For example, approximately 2 million tons of concrete wastes are generated annually in China (Xiao et al., 2012). The global construction and demolition waste reached more than 3.0 billion tons annually and is increasing constantly (Akhtar and Sarmah, 2018). Handling such a large amount of waste has become a serious problem. In addition, lots of nations in the word are facing shortages of natural sand and gravel resources, the acquisition of which has posed serious threats to the environment and society. Therefore, recycling construction waste as aggregates to address the demand for natural sand and gravel has become an effective method of concrete waste disposal. Green production of cement concrete or asphalt concrete using recycled concrete aggregate (RCA) can reduce construction costs, eliminate environmental pollution, and mitigate natural aggregate depletion (Bru et al., 2014; San Nicolas and Provis, 2015; Kareem et al., 2018; Fernández-Jiménez et al., 2019; Chen et al., 2020).
Extensive investigations have been conducted worldwide on the mechanical properties, durability, and structural performance of recycled aggregate concrete (Behera et al., 2014; Li et al., 2017; Akhtar and Sarmah, 2018; Khern et al., 2020). Old mortar attached to RCA is the most significant difference between concrete containing RCA and that containing natural aggregate, and is generally the main reason why the former is less desirable than the latter (Li et al., 2016; Lei et al., 2018). Several studies and engineering applications have shown that even with the same proportions, the performance of concrete with RCA is lower than that of natural aggregate; however, after reasonable mixture design or strengthening treatment, the concrete with RCA meets the requirements of strength and durability (Ajdukiewicz and Kliszczewicz, 2002; Pedro et al., 2017). Many modification methods have been explored to improve the strength and durability of recycled aggregate concrete. These methods can be divided into two categories: strengthening recycled aggregate concrete integrally with the addition of admixtures such as fly ash (Li et al., 2018) and nano-SiO2 (Mukharjee and Barai, 2014), and reinforcing RCA by treatment with a nanomaterial solution (Zhang H. et al., 2016) or polymer emulsion (Kou and Poon, 2010). The methods of reinforcing RCA in cement concrete cannot be used for asphalt mixtures, mainly because soaking in an acid and sodium silicate solution would reduce the cohesion between the RCA and the asphalt (Hou et al., 2014).
Several studies have been conducted on the potential usage of RCA in pavements. The mechanical properties of hot mixed asphalt (HMA) with RCA are usually inferior to those of HMA with natural coarse aggregate (NCA), which in turn affects the pavement performance of the HMA. With an increase in RCA content, the bulk specific gravity of the asphalt mixture decreases, whereas the optimum asphalt content (OAC) increases (Mills-Beale and You, 2010; Zhang Z. et al., 2016; El-Tahan et al., 2018). Mills-Beale and You (2010) reported that an increase in RCA content increased the resilient modulus but decreased the dynamic modulus. El-Tahan et al. (2018) reported that the incorporation of RCA in HMA led to an increase in stability, flow, and voids infilled with asphalt. Conversely, the bulk density, air voids, and voids in the mineral aggregate decreased. Zhang Z. et al. (2016) reported that as the RCA replacement levels increased, the high-temperature stability and low-temperature cracking resistance of the asphalt concrete generally increased. Moreover, they found that the water stability was significantly lower than that of asphalt concrete produced with NCA and that asphalt concrete containing up to 50% RCA can be satisfactorily used in road construction. Qasrawi and Asi (2016) reported that an increase in the RCA percentage decreased the resilient modulus of asphalt mixtures and increased their skid resistance. However, the use of 60/70 bitumen increased the resilient modulus and skid resistance of the asphalt mixtures compared with the use of 80/100 bitumen. Mixtures containing RCA showed lower water sensitivity (stripping resistance) than RCA-free mixtures, and bitumen 80/100 showed better performance for water sensitivity than bitumen 60/70.
Given the weaker nature of RCA materials, different methods of improving the pavement performance of asphalt concrete by treating the RCA have gained increasing popularity in recent years. Coating RCA with a 5% bitumen emulsion and waiting for the bitumen emulsion to break prior to the HMA mixing process improves the water resistance of the mixtures produced with RCA (Pasandín and Pérez, 2014). Using slag cement paste for pre-coated RCA can reinforce its ability to resist crushing and friction, and enhance the engineering properties of HMA containing RCA (Lee et al., 2012). Compared with using untreated RCA, RCA treated with hydrated lime effectively reduces the deterioration degree, and the improvement is amplified with high RCA usage rates. Both moisture susceptibility and fatigue life increase with the RCA usage rate, and incorporating treated RCA produces better results than using untreated RCA (Albayati et al., 2018). Cement-stabilized RCA treated with waste oil and asphalt emulsion showed satisfactory performance in strength, stiffness, and shrinkage properties (Zhou et al., 2019). Double coating technology (DCT) using slag paste and Sika Tite-BE can improve the strength and durability of RCA. In particular, DCT decreases the water absorption of double coated RCA (DCRCA) by 12.3 and 26.1% compared with uncoated RCA and RCA coated with cement slag paste, respectively, which effectively enhances the moisture resistance and stiffness of HMA (Kareem et al., 2018).
Modification of the asphalt binder is another approach for improving the pavement performance of asphalt concrete. Previous research has revealed that increasing the aging time of HMA containing RCA increased the number of air voids, the stiffness at ambient temperature, and the initial permanent deformation (Pasandín and Pérez, 2014). Polymer modifiers such as styrene-butadiene-styrene (SBS) and polyolefin crumb rubber or fibers are used as reinforcement in asphalt mixtures (Wang et al., 2018). Moreover, using fibers as reinforcement in asphalt mixtures for improving the asphalt pavement performance is becoming increasingly popular (Abtahi et al., 2010). Fibers provide multiple reinforcing and bridging functionalities and enable asphalt mixtures to withstand high temperatures and to minimize shearing deformation and low-temperature cracking. Further, fatigue failure, water damage, and freezing/thawing cycle resistance are significantly improved with the addition of fibers (Wu et al., 2008; Bonica et al., 2016; Jaskuła et al., 2017; Qin et al., 2018). According to Bentur and Mindess (2006), hybrid fibers can optimize the mechanical properties and durability of cementitious composites because they contain different types and sizes of fibers.
Permeable asphalt mixture (PAM) has advantages such as good traffic safety performance, noise reduction, drainage and flood control, and environmental protection (Coleri et al., 2013; Liao et al., 2014; Song et al., 2015). However, although PAM has many advantages in traditional compact asphalt concrete, its performance and service life are reduced (Xu et al., 2016; Zhang et al., 2018b). High-quality aggregate is an important factor for controlling the performance of PAM (Herndon et al., 2016). Crumb rubber modifier or the combination of fibers and SBS are the most effective components for increasing the abrasion resistance of PAM (Lyons and Putman, 2013). However, limited research has been conducted thus far involving RCA. Chen and Wong (2013) concluded that PAM composed of 100% RCA combined with asphalt modified by specific additive TAFPACK-Super can adequately fulfill the Marshall criteria of Singapore’s Land Transport Authority Standard stipulated for normal roads, indicating its potential use in highway applications. However, further research should be conducted to evaluate its feasibility for application to high-traffic highways.
Permeable asphalt mixture with RCA not only conforms to the trend of building sponge cities, but also alleviates the problem of overexploitation of natural aggregate resources. The purpose of this study is to explore the engineering properties of modified PAM with RCA, which is strengthened by two methods: (1) impregnating the RCA with three types of solutions including cement slurry, silicone resin emulsion, and a saturated calcium hydroxide solution, and (2) adding two types of fibers to asphalt: polyester and basalt. These findings provide a theoretical basis for promoting the applications of RCA for PAM in concrete pavement applications.
Experimental Program
Raw Materials
In this study, the SBS-modified asphalt was used, which is widely employed in PAM. Its basic parameters include penetration of 50.2 mm, softening point of 80.7°C, and ductility of 26.4 cm. The technical performances of basalt fine aggregate and mineral powder met the requirements of CJJ/T190 CAU (2012) (Technical Specification for Permeable Asphalt Pavement). The recycled coarse aggregate used in the test came from the concrete waste produced by the demolition of old houses. After hand breaking, the concrete waste was crushed by the jaw crusher in the laboratory, and then the aggregate within the range of 4.75–13.2 mm was screened and selected as shown in Figure 1. The gradation of aggregates was designed to be similar to PAM specimens with different proportions, which can meet the requirement of the standard by manual mixing, as shown in Figure 2. Compared with recycled fine aggregate with high water absorption and poor properties, recycled coarse aggregates are more suitable for use. At this stage, it would also be too complex to investigate and analyze if both the coarse and fine aggregates were treated. Thus, the authors decided to only treat the coarse aggregate, and use natural sand as the fine aggregate. RCA and treated RCA were used to replace NCA by 30, 50, and 100% in volume. For aggregates treated, these were taken randomly. After treatment, they were evenly mixed with other aggregates. Commercial silicone resin emulsion at a concentration of 50% and calcium hydroxide solution at a concentration of 1 mol/L were used to treat the RCA. The properties of the mineral filler are listed in Table 1. P.O. 42.5 Portland cement was used as the cement slurry for treating RCA; its chemical composition is listed in Table 2. NCA with particle sizes 4.75–16.0 mm and natural fine aggregate with particle sizes 0.075–4.75 mm are made from a crushed granite source, as shown in Figure 1. The properties of the NCA used in this study are summarized in Table 3. The length of the basalt and polyester fibers was 12 mm, as shown in Figure 1; their properties are listed in Table 4.
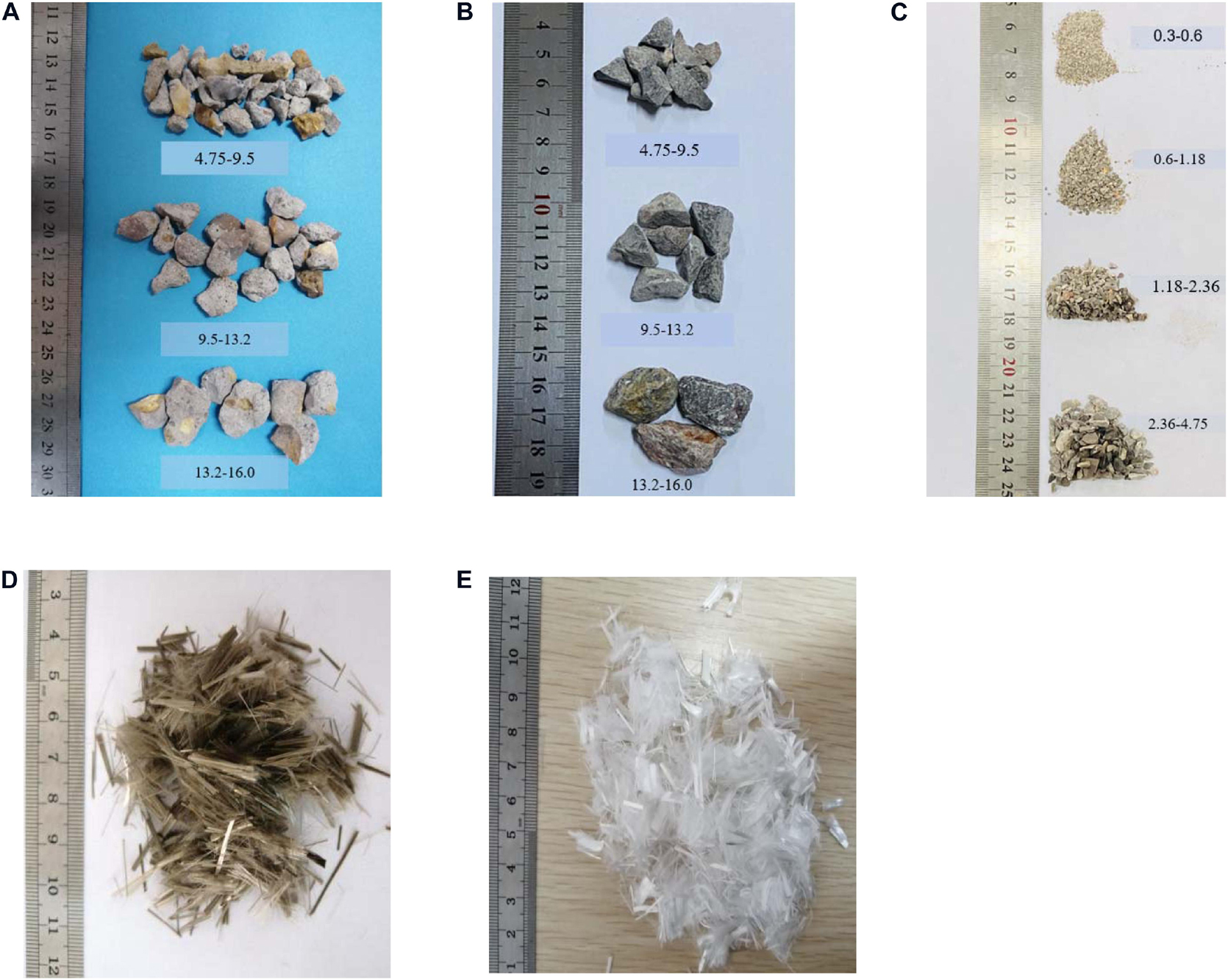
Figure 1. Morphology of aggregate and fibers. (A) Recycled coarse aggregate. (B) Natural coarse aggregate. (C) Natural fine aggregate. (D) Basalt fiber. (E) Polyester fiber.
Treatment of RCA
To improve the properties of PAM containing RCA, surface treatment by impregnating RCA was adopted by soaking the aggregate in separate treatments of cement slurry, silicone resin emulsion, and saturated calcium hydroxide solution. For treating RCA with the cement slurry (CSRCA), RCA with particle sizes larger than 4.75 mm was selected, washed, and dried. Then, cement slurry with a water-to-cement ratio of 0.5 was prepared in a container. The aggregate was poured into the container, screened out after shaking table application for 3 min, and then dried for 28 days, as shown in Figure 3A.
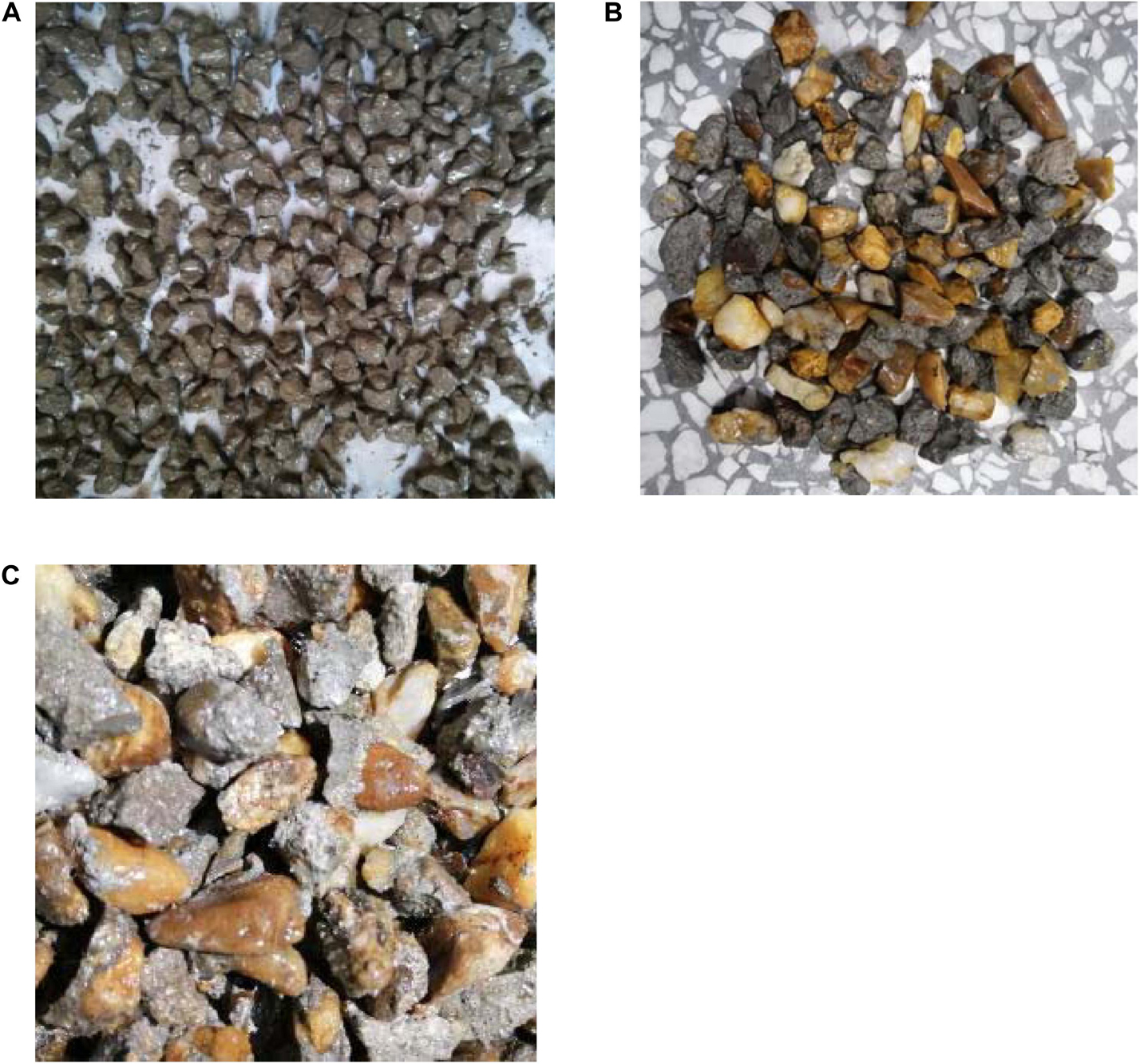
Figure 3. Different types of treatments for RCA. (A) Cement slurry. (B) Calcium hydroxide solution. (C) Silicone resin emulsion.
For treatment of the RCA with the calcium hydroxide solution (CHRCA), RCA with particle size larger than 4.75 mm was selected. The aggregate was then washed with water, soaked in a saturated calcium hydroxide solution for 2 days, and filtered out and dried for 28 days, as shown in Figure 3B. When treating RCA with the silicone resin emulsion (SRRCA), RCA with particle sizes of 4.75–9.5 mm and 9.5–13.2 mm were screened and cleaned, and the two aggregate types were separately treated. To ensure that the RCA surface was completely wrapped by the film formed by the silicone resin, the mass ratios of the silicone resin and RCA with particle sizes of 4.75–9.5 mm and 9.5–13.2 mm were 5.5 and 3%, respectively. This was diluted to 25% concentration in a bucket and was then thoroughly mixed with RCA and dried in an oven at 160°C for 4 h, as shown in Figure 3C.
PAM Preparation
In this test, PAM containing RCA with CSRCA, CHRCA, and SRRCA replacements of 30, 70, and 100% were manufactured. PAM with 100% RCA replacement and an added fiber content of 0.4% in mass percentage were also prepared, considering mass ratios of 1 to 0, 3 to 1, 2 to 2, 1 to 3, 0 to 1 between the basalt fibers and polyester fibers. The data of the PAM specimens are compiled in Table 4 and are labeled according to the coarse aggregate type, replacement ratio, and fiber content. For example, NCA represents PAM with only natural coarse aggregate, CSRCA70 represents PAM with a CSRCA content of 70% replacement ratio, and BPRCA22 represents PAM with a 2:2 mass ratio between the basalt fibers and polyester fibers and 100% RCA replacement. The specimens were all formed into standard Marshall blocks (101.6 mm in diameter and 63.5 mm in height) and were compacted by 50 blows applied to each side.
Test Methods
Measurement of Optimum Asphalt Content
Numerous methods are used for determining the OAC; among these, the Cantabro/draindown test and the Marshall test are the most widely used. In this study, a combination of the two methods was employed. First, the average value from the Cantabro and draindown tests was determined as the OAC. Then, the Marshall test was used to verify the accuracy of the OAC further. This was accomplished by selecting an asphalt mixture group with the asphalt content close to that identified by the previous method in order to identify the asphalt content with the maximum Marshall stability.
For the Cantabro test, the samples were first sorted to meet the sizes requirements 101.6 ± 0.2 mm in diameter and 63.5 ± 1.3 mm in height. Then, they were placed into a constant-temperature water tank after meeting set to 20°C. After 20 h, the surface water was wiped off by using a dry towel, and the mixture quality before the Cantabro test m0 was measured. The samples were then placed into a Los Angeles abrasion machine and were rotated for 300 revolutions at 30 rpm. The samples were removed, and the mixture quality after the Cantabro test m1 was measured. The Cantabro loss in asphalt mixture ΔS was calculated according to Eq. (1):
where ΔS is the Cantabro loss (%), m0 is the mixture quality before the Cantabro test (g), and m1 is the mixture quality after the Cantabro test (g).
The data on the specimens measured before and after the Cantabro test with different oil–stone ratios are shown in Figure 4. Four groups of experiments were conducted, and the average value was taken as the experimental result. For the draindown test, an 800 ml clean beaker was prepared and weighed as m0 at the start of the experiment. After mixing the asphalt and aggregate, 1000 g of the mixture was introduced in the beaker, and the total mass of beaker and mixture was weighed as m1. The beaker was covered with a glass cap, placed in an oven at 185°C, and removed after 1 h. With no vibration or external force applied, the beaker was turned upside down onto a glass plate and was weighed as m2. The following equation was used to calculate the percent of draindown:
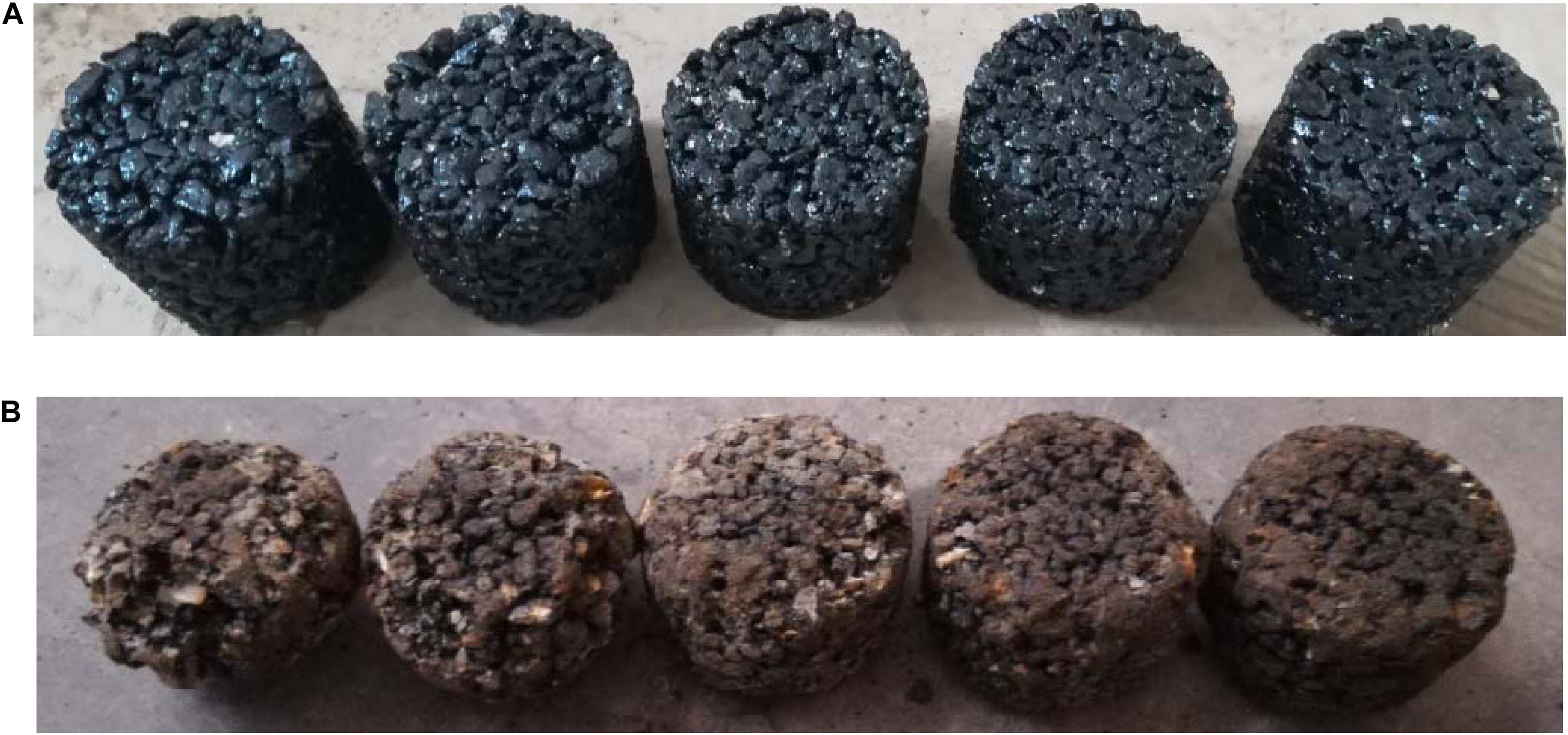
Figure 4. Permeable asphalt mixture before and after Cantabro test. (A) Before Cantabro test. (B) After Cantabro test.
where Δm is the percentage of draindown (%), m0 is the beaker quality before the experiment (g), m1 is the total quality of the beaker and asphalt mixture (g), and m2 is the quality of the beaker and residues after the experiment (g).
The draindown test was repeated four times, and the average value was taken as the final experimental result. Figure 5 includes a set of photographs captured after the draindown test. The value of OAC was obtained from the inflection point of the Cantabro loss curve and the inflection point of the percentage of the draindown curve, as shown in Figure 6. Then, the Marshall stability test was used to determine the OAC, as shown in Figure 7. Using RCA100 as an example, as shown in Figure 6D, the inflection point of the Cantabro loss curve and the percentage of the draindown curve were 7.1 and 7.6%; therefore, the asphalt content was initially determined to be their average value, i.e., 7.4%. Figure 7 shows that the Marshall stability of the mixture reached its maximum when the asphalt content was 7.4%. Therefore, the OAC of RCA100 was finally determined to be 7.4%.
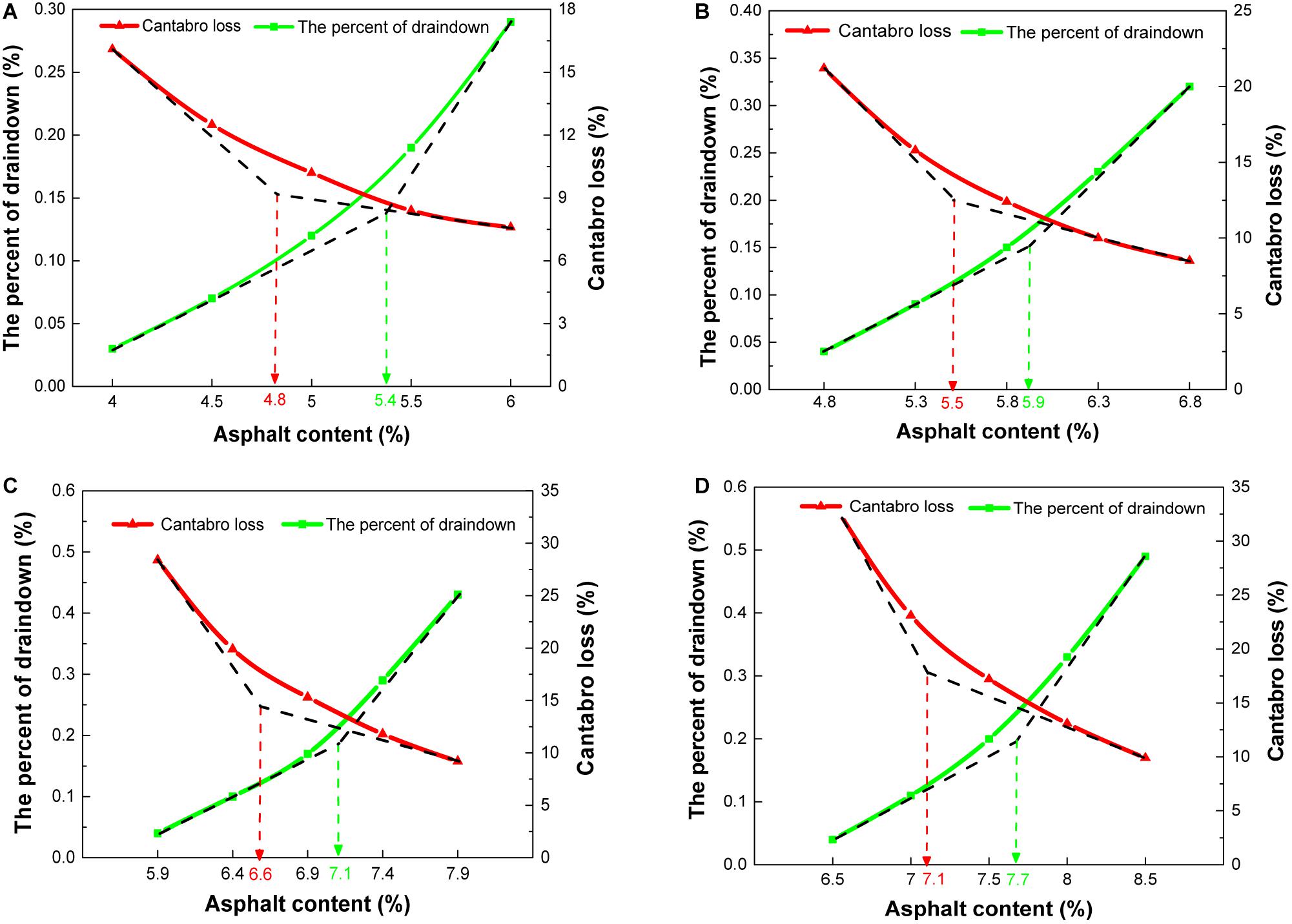
Figure 6. Cantabro loss in asphalt mixtures with different RCA content. (A) NCA; (B) RCA30; (C) RCA70; (D) RCA100.
Marshall Stability
The formula for calculating residual stability refers to JTJ 052 (2000) (Test Rules for Asphalt and Asphalt Mixture in Highway Engineering), as follows:
where MS0 is the residual stability of the mixture (%), MS1 is the immersion Marshall stability of the mixture (kN), and MS2 is Marshall stability of the mixture (kN).
The mixtures with different RCA contents were categorized into two groups, with four pieces included in each group. One group was placed into a constant-temperature water tank at 60°C for 30–40 min, and the stability and flow value were measured by using a Marshall testing machine. The second group was also placed into a constant-temperature water tank at 60°C but was held for 48 h to measure the immersion Marshall stability. Figure 8 shows images of the samples during different stages of the experiment.
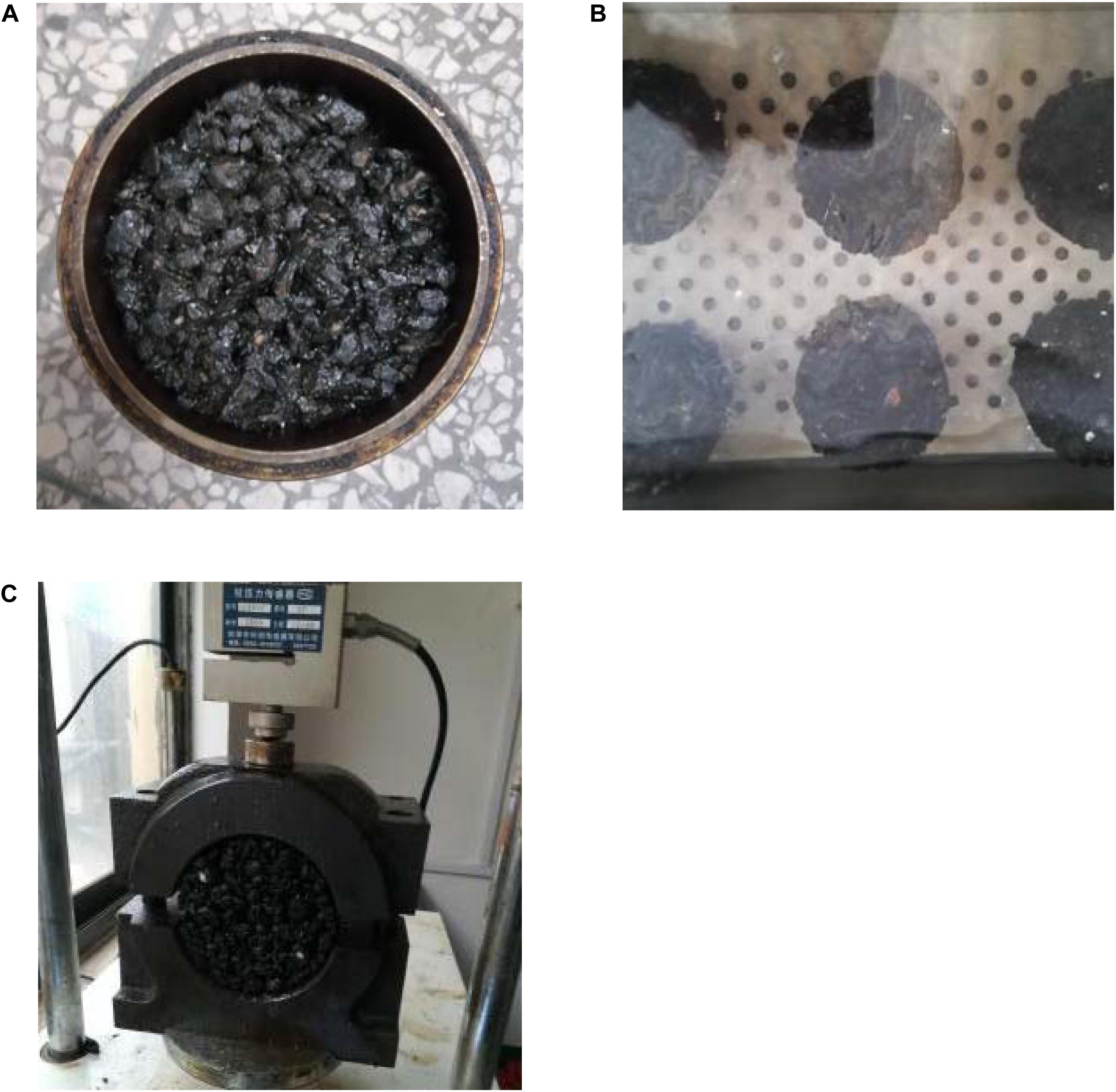
Figure 8. Process of Marshall test on PAM. (A) Compaction. (B) Immersion in water tank at constant temperature. (C) Measured stability.
Permeability
Permeable asphalt mixtures should have good water resistance and drainage capacity; therefore, it is necessary to measure its permeability coefficient and air voids. The air voids were measured following the technical specification of CJJ/T190 CAU (2012) (Technical Specification for Permeable Asphalt Pavement) (CJJ/T190 CAU, 2012). The permeability of PAM is tested under water head pressure in many studies (Gao et al., 2015). In reality, however, no water head pressure is usually present when the water is drained from the pavement. To simulate the actual drainage scenario as accurately as possible, a test method excluding water head pressure was adopted, as shown in Figure 9. First, the mixture was compacted in a sleeve die at a diameter of 101.6 mm. After cooling, the mixture, still in the mold, was immersed in water until becoming saturated. Finally, the mold was padded up and poured into 100 ml water. The time of complete water penetration (the end time of infiltration minus the start time) was recorded, and the permeability coefficient was calculated as follows:
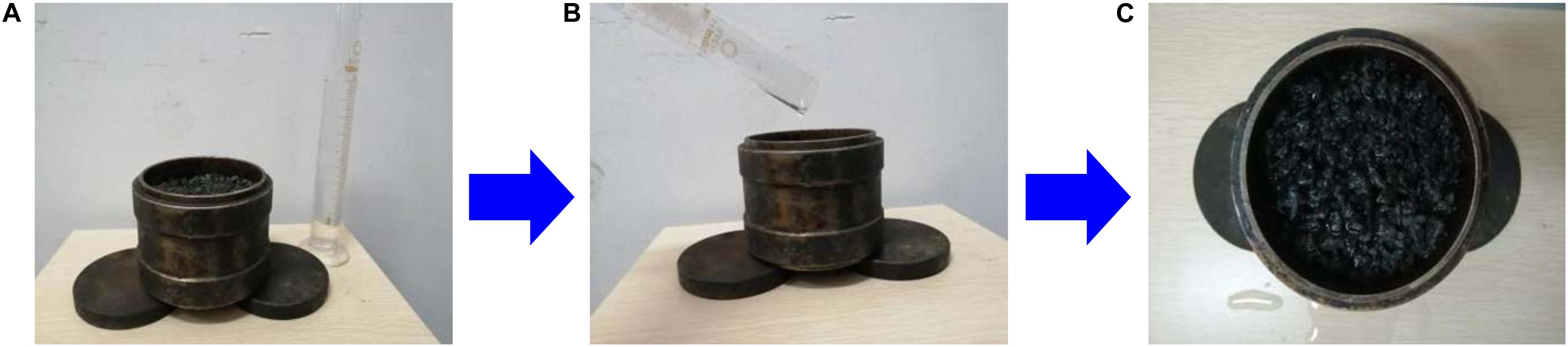
Figure 9. Permeability testing process on PAM. (A) Pad-up test block. (B) Pour water and mark the start time. (C) Mark the end time of infiltration.
where Q is the amount of water poured (ml), h is the specimen height (cm), 6.35 is the height of standard Marshall blocks (cm), A is the mixture area (cm2), and t is the osmotic time (s).
Results and Discussion
Performance of Coarse Aggregate
Chen and Wong (2013) found that the compressive strength of a porous asphalt mixture is adversely affected by the use of RCA to a larger degree than when using natural aggregate. The properties of coarse aggregate have a strong impact on the performance of the mixture. Table 5 shows the test results using various types of coarse aggregate. The water absorption rate and the crushing value of RCA were significantly higher than those of NCA, although the apparent density of the former was lower. This can be attributed to the significant amount of old cement mortar attached to the RCA surface, which has high water absorption and low strength. The water absorption rate and the crushing value of SRRCA showed obvious improvements even though the water absorption rate was slightly lower than that of NCA. This occurred because organic silicone resin forms a curing film on the surface of RCA (Hou et al., 2014), which improves its integrity and strength. Silicone resin itself is a hydrophobic material. Its water absorption is very low, and it prevents contact between water and the cement mortar, which effectively reduces the water absorption of the RCA. After soaking in the cement slurry and the calcium hydroxide solution, the water absorption and the crushing index of the RCA did not show significant differences compared with those measured in untreated RCA; these results are similar to those reported in a previous study (Zhang et al., 2018a). After the three surface treatments of RCA, the apparent density increased slightly. In particular, the adhesion level of the NCA and RCA to asphalt was 4, which meets the requirements of the adhesion level of coarse aggregate with asphalt listed in the technical specification of CJJ/T190 CAU (2012) (Technical Specification for Permeable Asphalt Pavement). After the three different surface treatment processes, the adhesion level of RCA increased to 5. This result indicates that the interface strength and the bonding force of the aggregate can be enhanced by surface treatment, which enables the asphalt cement to fully bond with the aggregate (Lee et al., 2012).
Optimum Asphalt Content of Asphalt Mixture
Effect of RCA Replacements on OAC
The OAC of mixtures with various RCA replacements is shown in Figure 10. The OAC increased with increases in the RCA replacements. The OAC of RCA100 was 45% higher than that of NCA. The asphalt easily entered the RCA after heating owing to the presence of several voids and cracks that developed during crushing and transportation. Moreover, the surface area of the old cement mortar was larger than that of NCA with the same volume. Therefore, the interface with the asphalt was larger, which led to an increase in the asphalt content (Qasrawi and Asi, 2016). The OAC of the mixture changed slightly after the treatment with the calcium hydroxide solution and the cement slurry. However, that of the mixture treated with silicone resin was significantly reduced. Specifically, the OAC of SRRCA100 was 16.2% lower than that of RCA100. Moreover, the OAC increased with the addition of fibers, as shown in Figure 10. The OAC of BPRCA10 and BPRCA01 increased by 2.7 and 10.8%, respectively, compared with RCA100. This occurred because the density of the polyester fibers is small, and those of the same mass have a large surface area, which facilitates absorption of additional asphalt (Lyons and Putman, 2013).
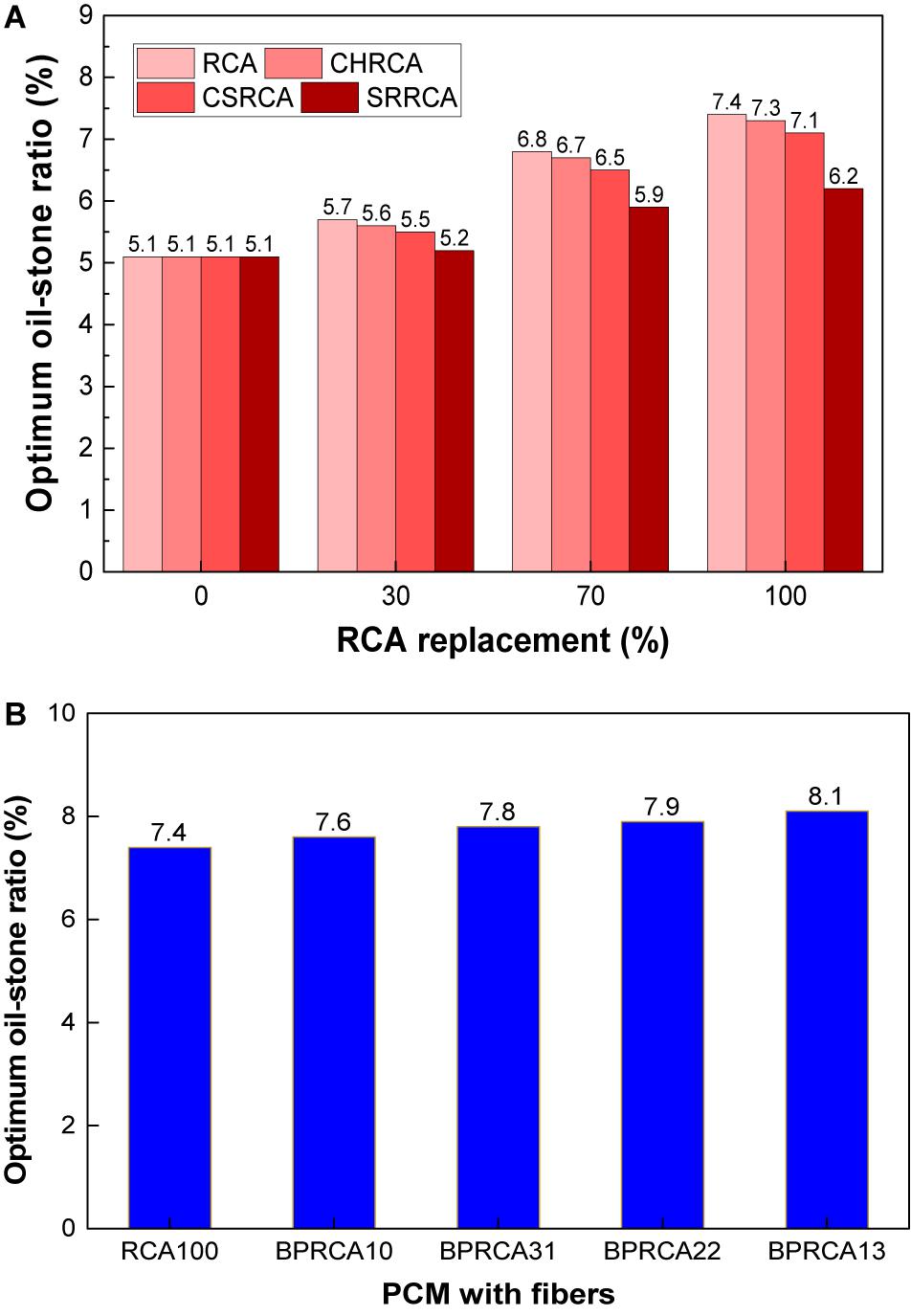
Figure 10. Effect of RCA treatment and the addition of fibers on the OAC of PAM. (A) RCA treatment. (B) Addition of fibers.
A one-way analysis of variance (ANOVA) test was conducted to examine the impact of RCA, CSRCA, CHRCA, and SRRCA dosage and fiber proportion on OAC of different mixtures. As shown in Table 6, these parameters had significant effects on the OAC at the 95% confidence level (p < 0.05). Therefore, changes in the CSRCA, CHRCA, and SRRCA dosage and fiber proportion affect the OAC of PAM.
Flow Value
The flow values of the different mixtures are shown in Figure 11. With an increase in RCA replacement, the flow value of asphalt mixture increased continuously, which indicates that plastic deformation increases with an increase in RCA replacement, as shown in Figure 11A. The flow value of RCA100 was 23.4% higher than that of NCA because the OAC increases with an increase in RCA replacement. The flow value of the asphalt mixture decreased after the RCA treatment; that with calcium hydroxide had the lowest value, followed those with silicone resin and cement slurry. Figure 11B shows that the flow value of the mixture increased with the addition of fibers; the increase of polyester fibers was caused by an increase in the OAC (Klinsky et al., 2018).
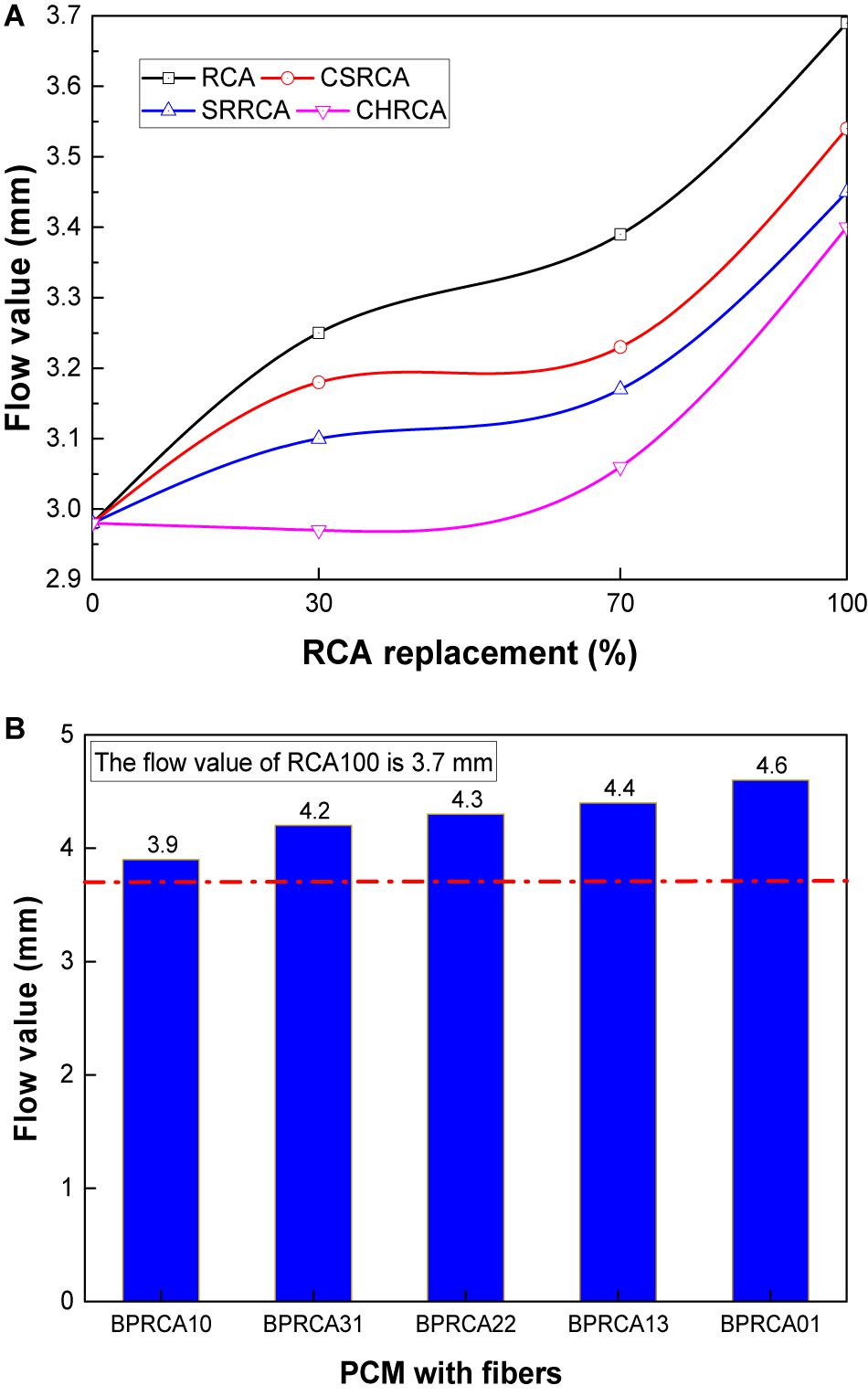
Figure 11. Effect of RCA treatment and the addition of fibers on the flow value of PAM. (A) RCA treatment. (B) Addition of fibers.
A one-way ANOVA test was conducted to examine the impact of RCA, CSRCA, CHRCA, and SRRCA dosage and fiber proportion on the flow value of the different mixtures, as listed in Table 6. The results showed that the RCA dosage and fiber proportion had significant effects at the 95% confidence level (p < 0.05). However, the effects of CSRCA, CHRCA, and SRRCA dosage were not significant at the 95% confidence level (p > 0.05). Thus, according the ANOVA results, the RCA dosage and fiber proportion have a greater effect than the CSRCA, CHRCA, and SRRCA dosage on the plastic deformation of the PAM flow value. Therefore, treating the RCA can reduce the plastic deformation in PAM.
Water Stability
Effect of Aggregate Content
The Marshall stability, immersion Marshall stability, and residual stability of PAM with different contents of various coarse aggregate are shown in Figure 12. The Marshall stability and immersion Marshall stability of PAM decreased with an increase in the content of RCA, CHRCA, and SRRCA, except for that with an RCA content of 70%. Specifically, the Marshall stabilities of RCA30, RCA70, and RCA100, were 11.2, 7.7, and 12.4% lower than those of NCA, respectively; the immersion Marshall stabilities of RCA30, RCA70, and RCA100 were 13.5, 13.8, and 22.3% lower than those of NCA, respectively; and the residual stabilities of RCA30, RCA70, and RCA100 were 2.5, 6.5, and 11.2% lower than those of NCA, respectively. This means that the strength of PAM decreases with an increase in the content of RCA, CHRCA, and SRRCA. However, in the case of CSRCA, the Marshall stability of PAM increased with an increase in the CSRCA content, and the immersion Marshall stability of PAM initially decreased slightly and then increased. This indicates that treating RCA with cement slurry greatly enhances the strength of PAM when the CSRCA content is high.
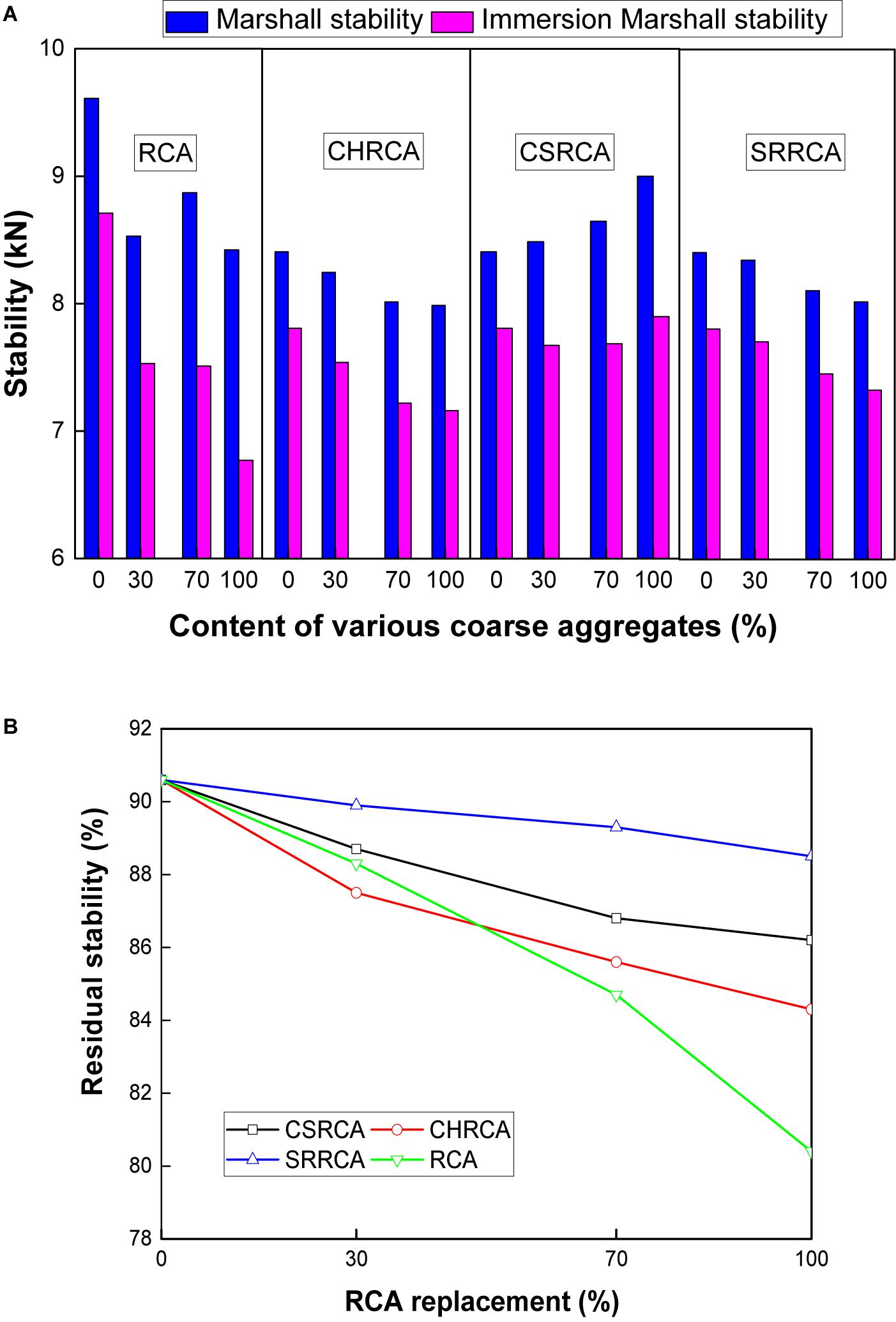
Figure 12. Marshall stability, immersion Marshall stability, and residual stability of PAM with different contents of various coarse aggregates. (A) Marshall stability and immersion Marshall stability (0% is concrete with just NCA). (B) Residual stability of permeable asphalt mixture.
Figure 12B further shows that the residual stability of PAM decreases with an increase in the content of RCA, CHRCA, CSRCA, and SRRCA because the RCA surface was coated with old cement mortar. Old cement mortar has low strength and is easily loosened and detached under pressure, which affects its integrity and strength. At the same time, many pebbles are present in RCA because the virgin aggregate is in this form. The pebble surface is acidic, leading to poor adhesion between the pebbles and asphalt (Zhang Z. et al., 2016). Moreover, after mixing the RCA and asphalt, the number of interfaces increased, which led to increases in the number of weak surfaces. Therefore, the addition of RCA has a strong influence on the strength and water stability of PAM. With an increase in the RCA content, the water stability of the mixture gradually decreased. When the RCA replacement was 100%, the residual stability of the mixture was only 80.4%, which is, at 80%, very close to the requirement of JTJ 052 (2000) (Test Rules for Asphalt and Asphalt Mixture in Highway Engineering). Therefore, to increase the residual stability of this proportion, it is necessary to either modify the RCA or enhance the PAM as a whole. A one-way ANOVA test was conducted to investigate the effect of replacement of the various coarse aggregates on the residual stability of PAM, as listed in Table 6. The results indicate that the replacement had a significant effect on the residual stability of PAM at the 95% confidence level (p = 0.009 < 0.05). Therefore, any changes in the content of RCA, CHRCA, CSRCA, or SRRCA also change the resistance of the produced mixture to moisture damage.
Effect of RCA Treatment
The Marshall stability, immersion Marshall stability, and residual stability of PAM with treated RCA are also shown in Figure 12. All decreased with an increase in CSRCA and SRRCA replacements, although they were still higher than that of RCA with the same replacement. This means that the strength and water stability of the mixture increased. For example, compared with that of RCA100, the Marshall stability of CSRCA100 and SRRCA100 increased by 6.7 and 7.2%, respectively; the immersion Marshall stability increased by 14.3 and 18.0%, respectively; and the residual stability increased by 7.2 and 10.1%, respectively. For CSRCA, the RCA used in this experiment contained pebbles, and the adhesion between pebbles and asphalt is poor. Because of the chemical adsorption between the acidic and alkaline cement slurry on the pebble surface, the cohesive force between the pebbles and the cement slurry increased. In addition, cement slurry can enter and fill the pores of RCA, which increases its strength and integrity (Lee et al., 2012). When the silicone resin treatment was adopted, the resin formed a solidified film on the surfaces of the aggregate and filled the pores of RCA, which increased the strength of RCA after curing at high temperature. The film wraps around the old mortar in RCA to prevent it from detaching, which further enhanced its integrity and strength (Shi et al., 2016).
With an increase in CHRCA replacement, the Marshall stability of the mixture increases, the immersion Marshall stability first decreased and then increased, and the residual stability of the mixture decreased. Compared with RCA100, the Marshall stability, immersion Marshall stability, and residual stability of CHRCA100 were 24.7, 30.7, and 4.9% higher than that of NCA, respectively. This indicates that RCA treated by the calcium hydroxide solution greatly enhanced the strength of PAM to a larger degree than that of NCA; however, its effect on the water stability of the mixture was not obvious. The RCA treated by silicone resin greatly improved the water stability of PAM to be very close to that of PAM with NCA; however, the influence on the strength of the mixture was not obvious. The RCA treated by the cement slurry improved both the strength and water stability of PAM; however, the improvement was not as great as the improvement after silicone resin treatment. When treated with a calcium hydroxide solution, the compound enters the crevices and voids of the aggregate. After contacting with air, calcium hydroxide reacts with carbon dioxide to form solid calcium carbonate, which can fill the voids and improve the strength of the aggregate (Albayati et al., 2018).
Effect of Fibers
For studying the influence of fibers on the stability of PAM, the RCA replacement ratio of all mixtures was 100%. The results of Marshall stability, immersion Marshall stability, and residual stability of mixtures with different fiber mixing methods are shown in Figure 13. All increased with the addition of fibers as shown in Figure 13A. For example, the Marshall stability of BPRCA10, with 0.4% basalt fiber, and BPRCA01, with 0.4% polyester fiber, was 32.3 and 3.1% higher than those of RCA100, respectively. In addition, the immersion Marshall stability of BPRCA10 and BPRCA01 was 43.3 and 18.9% higher than that of RCA100, respectively. Figure 13B also shows that the residual stability of BPRCA10 and BPRCA01 was 8.3 and 15.3% higher than that of RCA100, respectively. These values are similar to (or even higher than) that of NCA for the following reasons. First, fibers can absorb oil and resin in asphalt and increase the asphalt content, consistency (Xiang et al., 2018), and film thickness on aggregate surfaces, thus effectively preventing moisture damage at the asphalt and aggregate interface. Second, fibers change the interior of the asphalt binder into a network structure and increase the cohesion between the asphalt and the aggregate. With an increase in the polyester fiber content, the strength of the mixture decreases, and its water stability increases. For example, BPRCA10 had the highest Marshall stability and immersion Marshall stability but the lowest residual stability, whereas BPRCA01 had the lowest Marshall stability and immersion Marshall stability but the highest residual stability. This occurred because basalt fibers have a high tensile strength; thus, the strength of the mixture increases with increasing basalt fiber content. The density of polyester fiber is lower than that of basalt fiber, and the volume of polyester fiber is larger than that of basalt fiber at the same mass. In addition, the oil absorption rate of polyester fiber is larger than that of basalt fiber, which enables the polyester to absorb greater amounts of light components in the asphalt (Dai et al., 2018). Therefore, the water stability of PAM is improved by the addition of low-strength polyester fiber to a greater degree than by high-strength basalt fiber.
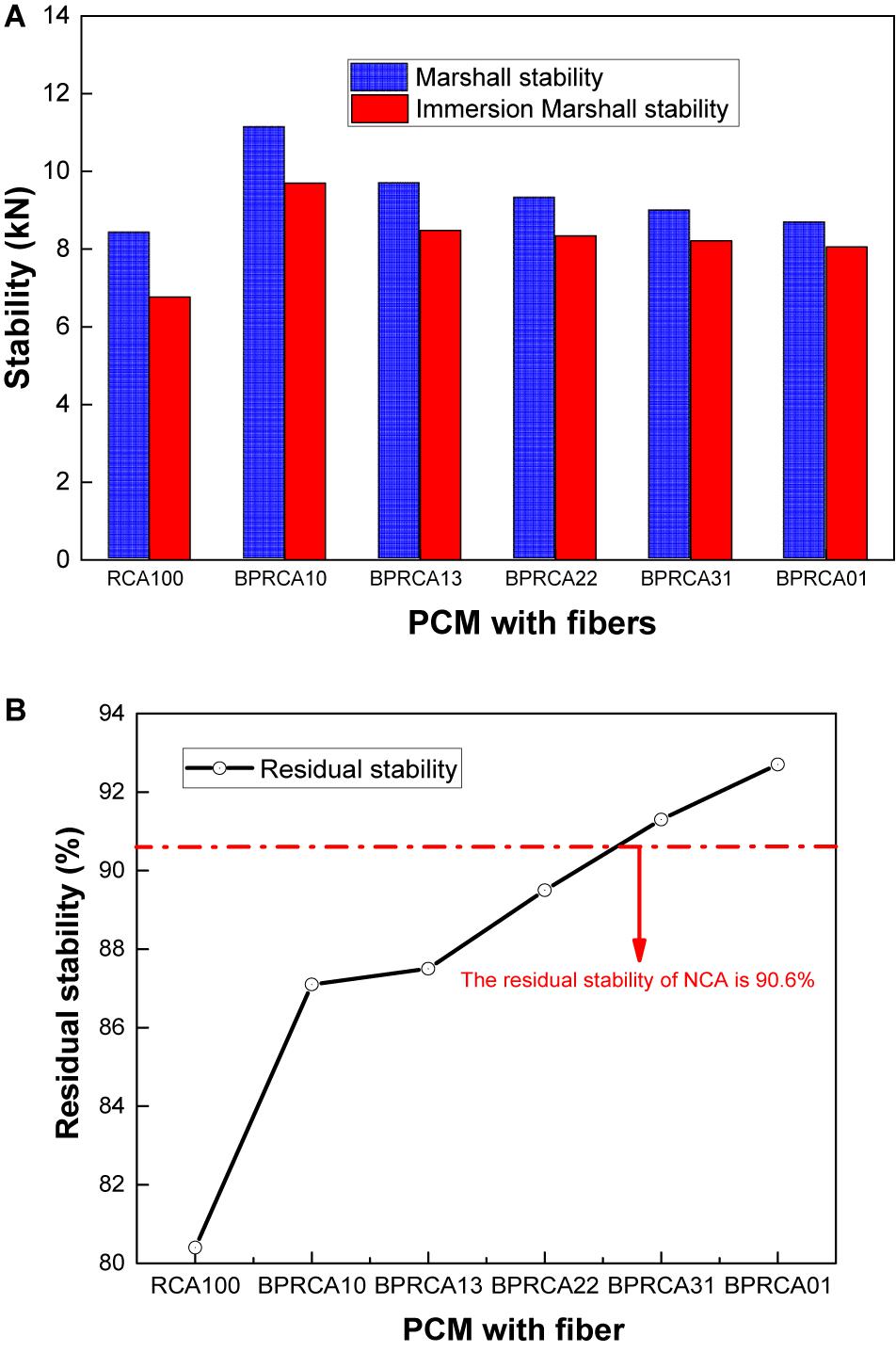
Figure 13. Effect of the addition of fibers on the Marshall stability, immersion Marshall stability, and residual stability of PAM. (A) Marshall stability, immersion Marshall stability. (B) Residual stability.
A one-way ANOVA test was conducted to examine the impact of CSRCA, CHRCA, and SRRCA dosage and fiber proportion on the residual stability of the different mixtures, as listed in Table 6. All had significant effects at the 95% confidence level (p < 0.05). Thus, any change in the CSRCA, CHRCA, and SRRCA dosage and fiber proportion produces a change in the residual stability of PAM.
Permeability
Previous studies have shown that the aggregate properties and the addition of fibers have little effect on the permeability of PAM (Sriravindrarajah et al., 2012; Afonso et al., 2017). The results of PAM mixtures with RCA are used here as a representative case to evaluate the permeability of the materials. The permeability coefficient and void volume test results of PAM with different RCA replacements are shown in Figure 14. The permeability coefficient and connected voidage of PAM decreased and then increased with an increase in RCA replacement. The change trend of the void fraction and permeability coefficient of mixtures was the same; the connected voidage and permeability of the mixture were positively correlated (Richardson, 1997). The permeability coefficients of RCA30 and RCA70 were 6.3 and 15.6% lower than those of NCA, respectively, because the OAC increases with an increase in RCA replacement, which decreases the number of connected voids. However, the permeability coefficient of RCA100 was 15.6% higher than that of NCA because more connected voids were created; similar conclusions were reported by Chen and Wong (2013).
The permeability coefficients of the mixtures with different RCA contents were greater than 0.01 cm/s, which is required in the specifications of CJJ/T190 CAU (2012) (Technical Specification for Permeable Asphalt Pavement). Therefore, it can be considered that the addition of RCA does not affect its drainage capacity. A one-way ANOVA test was conducted to investigate the effect of RCA dosage on the permeability of PAM, as listed in Table 6. The percentage of RCA did not significantly affect the permeability of PAM at the 95% confidence level (p = 0.657 > 0.05). Therefore, any change in the RCA dosage does not produce a change in the permeability of the produced mixture.
Disadvantages and Issues
Although the methodology is acceptable, the compaction method and the tests used are relatively simple and empirical (Marshall, Cantabro), which do not adequately represent in situ performance. There could be differences between the in situ performance and the test results from the laboratory. The constraints of the Marshall test mold on the asphalt mixture are different from the stress conditions of the pavement materials. Marshall’s impact compaction method is not conducive to the directional rearrangement of aggregates, resulting in the lower density of the mixture and the higher void ratio.
This study gives some preliminary exploratory test results by using RCA in PAM. The OAC and some parameters of permeable asphalt mixture are obtained. However, those test results are not enough to evaluate its application in the permeable pavement. Since durability and stability are also important aspects, further research such as performance at low temperatures and high temperatures, fatigue and rutting resistance are needed.
The results show that the OAC increased about 20–45%, and the cost of using RCA in PAM increased as compared with using normal aggregates. However, that of the mixture treated with silicone resin was significantly reduced. Specifically, the OAC of SRRCA100 was 16.2% lower than that of RCA100. It should also be noted that there are some limitations of RCA impregnation treatments, especially in terms of cost and practical handling issues, which highlights the necessity of relevant study in the future. If the source of construction waste can be controlled, it is possible to reduce the cost of asphalt aggregate selection for RCA to make RCA much cheaper than natural aggregate. As a consequence, the cost of PCM can be partially compensated. Besides, efforts could be made to optimize mix design and use low RCA content such as 30% to decrease OCA, making it more economical in practice.
This study is an innovative attempt to use modification treatments and hybrid fibers addition as two enhancement methods to improve the performance of PAM with RAC to be comparable to natural aggregate. It provides a new method for the efficient utilization of construction waste. It does need improvement with further research, but positive aspects of this study can benefit the field.
Conclusion
In this study, the properties of PAM with RCA replacements of 0, 30, 70, and 100% using various enhancement methods are investigated to satisfy the criteria for use in concrete pavement applications. The related conclusions are summarized in the following:
(1) The water absorption and crushing value of RCA are much higher than those of NCA. RCA treatment with silicone resin can dramatically reduce the absorption and crushing value of the RCA, and its water absorption rate becomes slightly lower than that of NCA. Although treating RCA with cement slurry and calcium hydroxide is unlikely to have a significant effect on the water absorption and crushing index, the adhesion level is enhanced from level 4 to level 5 after three different surface treatment processes.
(2) The OAC increases with an increase in RCA content. When the RCA replacement is 100%, the OAC increases 45%. After treated with calcium hydroxide solution or cement slurry, the OAC of the mixture changes slightly. However, the OAC of the mixture treated with silicone resin is significantly reduced by 16.2% compared with RCA100. The addition of fibers slightly increases the OAC.
(3) With an increase in RCA content, the residual stability of the mixtures decreases. When the RCA content is 100%, the residual stability of PAM decreases by 11.2% compared with PAM with NAC. RCA treatment by the calcium hydroxide solution greatly enhances the strength of PAM to a larger degree than that of NCA; however, its effect on the water stability of the mixture is not obvious.
(4) RCA treatment by silicone resin greatly improves the water stability of PAM to a degree very close to that of PAM with NCA; however, its influence on the strength of the mixture is not obvious. RCA treated by the cement slurry improves both the strength and water stability of PAM; however, the improvement is not as effective as that after silicone resin treatment. With an increase in RCA content, the permeability coefficient of PAM first decreases and then increases. The permeability coefficients of PAM with RCA meet the technical specifications.
(5) The ANOVA results indicate that the RCA, CSRCA, CHRCA, and SRRCA dosage and fiber proportion have significant effects on the OAC and the residual stability of PAM at the 95% confidence level (p < 0.05). The RCA dosage and fiber proportion had a stronger effect than the CSRCA, CHRCA, and SRRCA dosage on the plastic deformation of PAM flow value. The RCA dosage does not significantly affect the permeability of PAM.
Data Availability Statement
The raw data supporting the conclusions of this article will be made available by the authors, without undue reservation.
Author Contributions
BL and ZL: formal analysis, methodology, validation, and writing – original draft, review, and editing. WL: conceptualization, investigation, writing – original draft, review, and editing, funding acquisition, supervision, and resources. VT and KW: conceptualization, data curation, formal analysis, investigation, methodology, software, supervision, validation, visualization, and writing – review editing. WD: formal analysis, investigation, and writing – review and editing. All authors contributed to the article and approved the submitted version.
Funding
This research was supported by the National Natural Science Foundation of China (51668045 and 51968046) and Australian Research Council (DE150101751, DP200100057, IH150100006, and IH200100010).
Conflict of Interest
The authors declare that the research was conducted in the absence of any commercial or financial relationships that could be construed as a potential conflict of interest.
Acknowledgments
The authors also acknowledge the support from University of Technology Sydney Research Academic Program at Tech Lab (UTS RAPT) and University of Technology Sydney Tech Lab Blue Sky Research Scheme. ZL would like to appreciate the support by the Australian Government Research Training Program Scholarship, Australia.
References
Abtahi, S. M., Sheikhzadeh, M., and Hejazi, S. M. (2010). Fiber-reinforced asphalt-concrete-a review. Constr. Build. Mater. 24, 871–877.
Afonso, M. L., Dinis-Almeida, M., and Fael, C. S. (2017). Study of the porous asphalt performance with cellulosic fibres. Constr. Build. Mater. 135, 104–111. doi: 10.1016/j.conbuildmat.2016.12.222
Ajdukiewicz, A., and Kliszczewicz, A. (2002). Influence of recycled aggregates on mechanical properties of HS/HPC. Cem. Concr. Compos. 24, 269–279. doi: 10.1016/s0958-9465(01)00012-9
Akhtar, A., and Sarmah, A. K. (2018). Construction and demolition waste generation and properties of recycled aggregate concrete: a global perspective. J. Clean. Prod. 186, 262–281. doi: 10.1016/j.jclepro.2018.03.085
Albayati, A., Wang, Y., Wang, Y., and Haynes, J. (2018). A sustainable pavement concrete using warm mix asphalt and hydrated lime treated recycled concrete aggregates. SM&T 18:e00081. doi: 10.1016/j.susmat.2018.e00081
Behera, M., Bhattacharyya, S., Minocha, A., Deoliya, R., and Maiti, S. (2014). Recycled aggregate from C&D waste & its use in concrete-A breakthrough towards sustainability in construction sector: a review. Constr. Build. Mater. 68, 501–516.
Bentur, A., and Mindess, S. (2006). Fibre Reinforced Cementitious Composites. New York: Taylor & Francis.
Bonica, C., Toraldo, E., Andena, L., Marano, C., and Mariani, E. (2016). The effects of fibers on the performance of bituminous mastics for road pavements. Compos. B Eng. 95, 76–81. doi: 10.1016/j.compositesb.2016.03.069
Bru, K., Touzé, S., Bourgeois, F., Lippiatt, N., and Ménard, Y. (2014). Assessment of a microwave-assisted recycling process for the recovery of high-quality aggregates from concrete waste. Int. J. Miner. Process. 126, 90–98. doi: 10.1016/j.minpro.2013.11.009
Chen, M. J., and Wong, Y. D. (2013). Porous asphalt mixture with 100% recycled concrete aggregate. Road Mater. Pavement Des. 14, 921–932. doi: 10.1080/14680629.2013.837839
Chen, Z., Zhou, J., Ban, M., and Wang, X. (2020). Residual bond behavior of steel reinforced recycled aggregate concrete after exposure to elevated temperatures. Front. Mater. 7:142. doi: 10.3389/fmats.2019.00142
CJJ/T190 CAU (2012). Technical Specification for Permeable Asphalt Pavement. Beijing: China Architecture and Building Press.
Coleri, E., Kayhanian, M., Harvey, J. T., Yang, K., and Boone, J. M. (2013). Clogging evaluation of open graded friction course pavements tested under rainfall and heavy vehicle simulators. J. Environ. Manag. 129, 164–172. doi: 10.1016/j.jenvman.2013.07.005
Dai, Z., Shen, J., Shi, P., Zhu, H., and Li, X. (2018). Nano-sized morphology of asphalt components separated from weathered asphalt binders. Constr. Build. Mater. 182, 588–596. doi: 10.1016/j.conbuildmat.2018.06.127
El-Tahan, D., Gabr, A., El-Badawy, S., and Shetawy, M. (2018). Evaluation of recycled concrete aggregate in asphalt mixes. Innov. Infrastruct. Solut. 3:20.
Fernández-Jiménez, A., Garcia-Lodeiro, I., Maltseva, O., and Palomo, A. (2019). Mechanical-chemical activation of coal fly ashes: an effective way for recycling and make cementitious materials. Front. Mater. 6:51. doi: 10.3389/fmats.2019.00051
Gao, J., Guo, C., and Liu, Y. (2015). Measurement of pore water pressure in asphalt pavement and its effects on permeability. Measurement 62, 81–87. doi: 10.1016/j.measurement.2014.11.013
Herndon, D. A., Xiao, F., Amirkhanian, S., and Wang, H. (2016). Investigation of Los Angeles value and alternate aggregate gradations in OGFC mixtures. Constr. Build. Mater. 110, 278–285. doi: 10.1016/j.conbuildmat.2016.01.008
Hou, Y., Ji, X., Su, X., Zhang, W., and Liu, L. (2014). Laboratory investigations of activated recycled concrete aggregate for asphalt treated base. Constr. Build. Mater. 65, 535–542. doi: 10.1016/j.conbuildmat.2014.04.115
Jaskuła, P., Stienss, M., and Szydłowski, C. (2017). Effect of polymer fibres reinforcement on selected properties of asphalt mixtures. Proc. Eng. 172, 441–448. doi: 10.1016/j.proeng.2017.02.026
Kareem, A. I., Nikraz, H., and Asadi, H. (2018). Evaluation of the double coated recycled concrete aggregates for hot mix asphalt. Constr. Build. Mater. 172, 544–552. doi: 10.1016/j.conbuildmat.2018.03.158
Khern, Y. C., Paul, S. C., Kong, S. Y., Babafemi, A. J., Anggraini, V., Miah, M. J., et al. (2020). Impact of chemically treated waste rubber tire aggregates on mechanical, durability and thermal properties of concrete. Front. Mater. 7:90. doi: 10.3389/fmats.2019.00090
Klinsky, L., Kaloush, K., Faria, V., and Bardini, V. (2018). Performance characteristics of fiber modified hot mix asphalt. Constr. Build. Mater. 176, 747–752. doi: 10.1016/j.conbuildmat.2018.04.221
Kou, S.-C., and Poon, C.-S. (2010). Properties of concrete prepared with PVA-impregnated recycled concrete aggregates. Cem. Concr. Compos. 32, 649–654. doi: 10.1016/j.cemconcomp.2010.05.003
Lee, C.-H., Du, J.-C., and Shen, D.-H. (2012). Evaluation of pre-coated recycled concrete aggregate for hot mix asphalt. Constr. Build. Mater. 28, 66–71. doi: 10.1016/j.conbuildmat.2011.08.025
Lei, B., Li, W., Tang, Z., Tam, V. W., and Sun, Z. (2018). Durability of recycled aggregate concrete under coupling mechanical loading and freeze-thaw cycle in salt-solution. Constr. Build. Mater. 163, 840–849. doi: 10.1016/j.conbuildmat.2017.12.194
Li, W., Long, C., Tam, V. W., Poon, C.-S., and Duan, W. H. (2017). Effects of nano-particles on failure process and microstructural properties of recycled aggregate concrete. Constr. Build. Mater. 142, 42–50. doi: 10.1016/j.conbuildmat.2017.03.051
Li, W., Luo, Z., Long, C., Wu, C., Duan, W. H., and Shah, S. P. (2016). Effects of nanoparticle on the dynamic behaviors of recycled aggregate concrete under impact loading. Mater. Des. 112, 58–66. doi: 10.1016/j.matdes.2016.09.045
Li, Y., Wang, R., Li, S., Zhao, Y., and Qin, Y. (2018). Resistance of recycled aggregate concrete containing low-and high-volume fly ash against the combined action of freeze-thaw cycles and sulfate attack. Constr. Build. Mater. 166, 23–34. doi: 10.1016/j.conbuildmat.2018.01.084
Liao, G., Sakhaeifar, M. S., Heitzman, M., West, R., Waller, B., Wang, S., et al. (2014). The effects of pavement surface characteristics on tire/pavement noise. Appl. Acoust. 76, 14–23. doi: 10.1016/j.apacoust.2013.07.012
Lyons, K. R., and Putman, B. J. (2013). Laboratory evaluation of stabilizing methods for porous asphalt mixtures. Constr. Build. Mater. 49, 772–780. doi: 10.1016/j.conbuildmat.2013.08.076
Mills-Beale, J., and You, Z. (2010). The mechanical properties of asphalt mixtures with recycled concrete aggregates. Constr. Build. Mater. 24, 230–235. doi: 10.1016/j.conbuildmat.2009.08.046
Mukharjee, B. B., and Barai, S. V. (2014). Influence of nano-silica on the properties of recycled aggregate concrete. Constr. Build. Mater. 55, 29–37. doi: 10.1016/j.conbuildmat.2014.01.003
Pasandín, A., and Pérez, I. (2014). Mechanical properties of hot-mix asphalt made with recycled concrete aggregates coated with bitumen emulsion. Constr. Build. Mater. 55, 350–358. doi: 10.1016/j.conbuildmat.2014.01.053
Pedro, D., De Brito, J., and Evangelista, L. (2017). Evaluation of high-performance concrete with recycled aggregates: use of densified silica fume as cement replacement. Constr. Build. Mater. 147, 803–814. doi: 10.1016/j.conbuildmat.2017.05.007
Qasrawi, H., and Asi, I. (2016). Effect of bitumen grade on hot asphalt mixes properties prepared using recycled coarse concrete aggregate. Constr. Build. Mater. 121, 18–24. doi: 10.1016/j.conbuildmat.2016.05.101
Qin, X., Shen, A., Guo, Y., Li, Z., and Lv, Z. (2018). Characterization of asphalt mastics reinforced with basalt fibers. Constr. Build. Mater. 159, 508–516. doi: 10.1016/j.conbuildmat.2017.11.012
Richardson, D. N. (1997). Drainability characteristics of granular pavement base material. J. Transp. Eng. 123, 385–392. doi: 10.1061/(asce)0733-947x(1997)123:5(385)
San Nicolas, R., and Provis, J. L. (2015). The interfacial transition zone in alkali-activated slag mortars. Front. Mater. 2:70. doi: 10.3389/fmats.2019.00070
Shi, C., Li, Y., Zhang, J., Li, W., Chong, L., and Xie, Z. (2016). Performance enhancement of recycled concrete aggregate-a review. J. Clean. Prod. 112, 466–472. doi: 10.1016/j.jclepro.2015.08.057
Song, W., Shu, X., Huang, B., and Woods, M. (2015). Factors affecting shear strength between open-graded friction course and underlying layer. Constr. Build. Mater. 101, 527–535. doi: 10.1016/j.conbuildmat.2015.10.036
Sriravindrarajah, R., Wang, N. D. H., and Ervin, L. J. W. (2012). Mix design for pervious recycled aggregate concrete. Int. J. Concr. Struct. Mater. 6, 239–246. doi: 10.1007/s40069-012-0024-x
Wang, J., Yuan, J., Xiao, F., Li, Z., Wang, J., and Xu, Z. (2018). Performance investigation and sustainability evaluation of multiple-polymer asphalt mixtures in airfield pavement. J. Clean. Prod. 189, 67–77. doi: 10.1016/j.jclepro.2018.03.208
Wu, S., Ye, Q., and Li, N. (2008). Investigation of rheological and fatigue properties of asphalt mixtures containing polyester fibers. Constr. Build. Mater. 22, 2111–2115. doi: 10.1016/j.conbuildmat.2007.07.018
Xiang, Y., Xie, Y., and Long, G. (2018). Effect of basalt fiber surface silane coupling agent coating on fiber-reinforced asphalt: from macro-mechanical performance to micro-interfacial mechanism. Constr. Build. Mater. 179, 107–116. doi: 10.1016/j.conbuildmat.2018.05.192
Xiao, J., Li, W., Fan, Y., and Huang, X. (2012). An overview of study on recycled aggregate concrete in China (1996-2011). Constr. Build. Mater. 31, 364–383. doi: 10.1016/j.conbuildmat.2011.12.074
Xu, B., Chen, J., Li, M., Cao, D., Ping, S., Zhang, Y., et al. (2016). Experimental investigation of preventive maintenance materials of porous asphalt mixture based on high viscosity modified bitumen. Constr. Build. Mater. 124, 681–689. doi: 10.1016/j.conbuildmat.2016.07.122
Zhang, H., Ji, T., Liu, H., and Su, S. (2018a). Modifying recycled aggregate concrete by aggregate surface treatment using sulphoaluminate cement and basalt powder. Constr. Build. Mater. 192, 526–537. doi: 10.1016/j.conbuildmat.2018.10.160
Zhang, H., Li, H., Zhang, Y., Wang, D., Harvey, J., and Wang, H. (2018b). Performance enhancement of porous asphalt pavement using red mud as alternative filler. Constr. Build. Mater. 160, 707–713. doi: 10.1016/j.conbuildmat.2017.11.105
Zhang, H., Zhao, Y., Meng, T., and Shah, S. P. (2016). Surface treatment on recycled coarse aggregates with nanomaterials. J. Mater. Civ. Eng. 28:04015094. doi: 10.1061/(asce)mt.1943-5533.0001368
Zhang, Z., Wang, K., Liu, H., and Deng, Z. (2016). Key performance properties of asphalt mixtures with recycled concrete aggregate from low strength concrete. Constr. Build. Mater. 126, 711–719. doi: 10.1016/j.conbuildmat.2016.07.009
Keywords: recycled concrete aggregate, permeable asphalt mixture, permeability, pavement, enhancement
Citation: Lei B, Li W, Luo Z, Tam VWY, Dong W and Wang K (2020) Performance Enhancement of Permeable Asphalt Mixtures With Recycled Aggregate for Concrete Pavement Application. Front. Mater. 7:253. doi: 10.3389/fmats.2020.00253
Received: 09 May 2020; Accepted: 13 July 2020;
Published: 16 September 2020.
Edited by:
Guangming Chen, South China University of Technology, ChinaCopyright © 2020 Lei, Li, Luo, Tam, Dong and Wang. This is an open-access article distributed under the terms of the Creative Commons Attribution License (CC BY). The use, distribution or reproduction in other forums is permitted, provided the original author(s) and the copyright owner(s) are credited and that the original publication in this journal is cited, in accordance with accepted academic practice. No use, distribution or reproduction is permitted which does not comply with these terms.
*Correspondence: Wengui Li, wengui.li@uts.edu.au